The image of technology marching forward to the beat of its own drum, sending repercussions throughout society, has been repeatedly and successfully challenged in the past decade. We have yet to persuade wider audiences, but at least in the general field of science and technology studies, technological determinism is dead.
The demise of technological determinism means that studies of technological design and development must now do more than just show how political, social, economic, and cultural considerations shape and become part of technology. We are presently poised to use technological artifacts as lenses through which to view broader historical questions, to understand how the process of shaping technology can also be the process of shaping politics, society, and culture. The time is ripe for the history of technological design and development to become a more integral part of mainstream history.1
This article delineates one way in which a close examination of technological design can contribute to broader historical questions. By placing the design of two nuclear reactors in the political framework of postwar France, I show that these reactors were more than technological artifacts. Engineers and managers inscribed their political agendas into the design of their reactors, and for a variety of reasons, including the instability of government leadership in the 1950s, these designs in turn became part of French political discourse. Each reactor came to embody a particular vision of the French state, and each became a powerful tool in shaping both nuclear and industrial policy in postwar France.2
The article begins with a quick sketch of postwar France and an outline of the creation of the two institutions that made up the core of the French nuclear program: the Commissariat à l’Énergie Atomique (CEA)—the atomic energy commission—and Électricité de France (EDF)—the nationalized electric utility company. Through the early 1950s, the nuclear program rested solely in the hands of CEA leaders, so I next discuss how these men picked the gas-graphite reactor design over other choices. Finally, the bulk of this article compares the designs of two early gas-graphite reactors, one financed by the CEA, the other by EDF. The two institutions collaborated on the design and construction of both reactors: France did not have the financial, human, or political resources to sustain separate programs. But, even though each institution needed the other in order to build a nuclear program, they had different, sometimes conflicting, political, industrial, and technological agendas. Each reactor thus became a distinct political, industrial, and technological statement.
2.1 France after the Second World War
The Second World War left France both economically and psychologically devastated. The German occupants had rendered more than half of the railroad network unusable, they had requisitioned most of the machine tools under twenty-five years of age, they had exported 15 percent of all agriculture products—and the list goes on. Almost as damaging, the French had suffered the third defeat at the hands of the Germans in seventy years—and this was by far the most mortifying, as it subjected them to four years of foreign occupation and the opprobrious spectacle of their fellow citizens collaborating in their humiliation.3
No surprise, then, that after the Liberation left-wing politicians and followers of Charles de Gaulle alike shared the pressing priority of reconstructing their nation’s economy and morale. Further, they shared the belief that France’s defeat resulted largely from the “economic feudalism” and “Malthusianism” practiced by private industry and politicians before the war. The state, they concluded, should provide the impulse for, as well as direct, the reconstruction. By engaging in and promoting investments aimed at modernizing and expanding French industry, the state would accomplish the dual aim of resuscitating the economy and restoring the country to its rightful place in the ranks of great nations.4
This reconstruction effort advanced on many fronts. A new government institution known as the Commissariat Général au Plan (the Planning Commission) set nationwide production goals and coordinated economic development in various private and public industrial sectors.5 The electricity, coal, and gas industries were nationalized, making the state the only shareholder in these companies. Thus, the new economic structure would, in principle at least, serve the French people rather than private interests.6
In order to ensure the success of this drive for industrial strength, politicians drew on a resource that France had had for almost two hundred years, the grands corps de l’état, and in particular on the two engineering corps, the Corps des Mines and the Corps des Ponts et Chaussées. These corps, made up almost exclusively of graduates of the École Polytechnique, had long espoused an ideology of engineering in the public service. Their power within the state administration, however, had waxed and waned over the years. In the I9th century, their engineers had built French railroads and exploited French mines.7 But their efforts to “rationalize” the state economy through planning during the Depression had failed because of their insistence on remaining above the “irrational” processes of politics.8 Postwar France saw a massive resurgence of grands corps engineers in positions of power in the ministries, on the Planning Commission, and at the head of the nationalized industries.
One such establishment was Électricité de France. Before the war, a multitude of private companies had supplied the nation’s electricity, using different networks that ran on different frequencies and voltages. To many members of the postwar provisional government, these companies represented the epitome of capitalist evil, privileging short-term profit making over providing coherent public service. Further, the heterogeneity of the distribution and transmission network undermined the reliability of the electricity supply. Engineers, labor unions, and politicians agreed that the new France should be on a single, standardized electrical network run by a single, nationalized institution. After heated debates on the precise ways in which such an institution should be structured, a nationalization law finally passed in April 1946. It regrouped the private companies into a single electric utility, EDF, which was accountable for its expenditures to the Ministry of Finance and for its development program to the Ministry of Industry. The new utility had a mission to provide France with a reliable, cheap, and abundant supply of electricity, and it immediately embarked on a massive hydroelectric program.9
Whereas EDF was the subject of much public discussion, the atomic energy commission grew out of backstage negotiations. After Hiroshima and Nagasaki, politician Raoul Dautry and Communist physicist Frédéric Joliot had no trouble convincing de Gaulle, the head of the postwar provisional government from 1945 to 1946, that a nuclear program would both elevate France’s stature in international politics and accelerate its industrial and economic recovery. Following de Gaulle’s recommendation, the National Assembly approved the creation of the Commissariat à l’Énergie Atomique in October 1945. The agency’s stated mission was to pursue “scientific and technical research with a view to the utilization of atomic energy in the several areas of science, industry, and national defense.”10 In order to fulfill this mission, the creation ordinance continued, “[The CEA has to be] very close to the Government and, so to speak, mingled with it, and nevertheless vested with great freedom of action. … It must be very close to the Government because the fate of the nation can be affected by developments in this branch of science, and it is therefore indispensable that it be under the authority of the Government. It must, on the other hand, be vested with great freedom of action because this is the sine qua non of its efficacy.”11
The CEA was the only public institution with such a high degree of autonomy, the only one not accountable to a specific ministry, and the only one not subject to the same financial controls as other state enterprises. Its internal organization reflected an ambiguous marriage of science and politics: it was a dyarchy headed by High Commissioner Joliot and Administrator General Dautry.
Both EDF and the CEA, then, grew out of the postwar vision of the kind of relationship between industrial development and the state that would ensure the reconstruction of the nation. Most high-level officials in each institution belonged to one of the elite engineering corps and had therefore been inducted into the great French tradition of engineering in the public service.12 In accordance with this tradition, these officials saw themselves as guardians of the public interest in matters technological. But EDF, as a nationalized company, reflected the left-wing side of the postwar coalition government, while the CEA, especially after Joliot’s departure in 1950, represented the Gaullist side.13 Officials in each institution, therefore, tended to have different definitions of the public interest. As we shall see, these definitions, more than any formal government decision, would determine the course of the nuclear program.
2.2 Negotiating a Design
In the late 1940s and early 1950s, atomic development rested solely with the CEA. Its scientists and engineers, therefore, set the initial parameters of the nuclear program. Initially, that program concentrated on locating uranium mines and building research reactors. Although “national defense” had been mentioned in the original ordinance, building an atomic bomb seemed out of the question, both technologically and politically. In its twelve years, 1946–58, the Fourth Republic saw over twenty different heads of state; for eleven out of those twelve years, the French representative to the United Nations asserted that France would never build a bomb. But, these statements did not suffice to soothe the United States in an atmosphere of intensifying Cold War. When the CEA’s high commissioner, Joliot, publicly declared in 1950 that he would not build a bomb because such a weapon could only be destined for the Soviet Union, American leaders protested the presence of a Communist at the head of such a strategically sensitive institution. In April 1950 Joliot was dismissed and replaced a year later by another eminent (but less vocal) scientist, Francis Perrin.14 In August 1951, the CEA’s administrator general Raoul Dautry died.
These developments left the CEA without apparent direction. It had found an important political ally, however, in parliamentary deputy Félix Gaillard.15 In 1951, Gaillard became a state secretary, in which capacity he served as the government’s official representative to the CEA’s steering committee.16 Convinced that France’s future lay in the strength of its nuclear program, he urged the committee to draft an ambitious five-year plan for the development of atomic energy—one that would seduce Parliament by promising material benefits not in fifty years, but in the near future. It would be easier, Gaillard said, to justify a 20-billion-franc plan that included developing atomic energy on an industrial scale than a 3-billion-franc plan devoted only to basic research.17 Perrin and other scientists expressed doubts about whether the CEA could carry out such an extensive program. But, technocrats such as François de Rose of the Ministry of Foreign Affairs supported Gaillard. The CEA should aim high, he said. True, France led the second-tier nuclear nations, but, he argued, this might change if Germany decided to start a large-scale nuclear program. In such an event, France’s future leaders would thank the CEA for having the foresight to plan extensive nuclear development.
Persuaded by these arguments, steering committee members agreed that the next step was to build a full-scale reactor. But what sort of reactor? Primary reactors, such as those built by the British, ran on natural uranium, of which France had plenty. So-called secondary reactors, developed in the United States, ran on enriched uranium. To run secondary reactors the CEA needed enriched uranium, which was not for sale anywhere. It would therefore have to build a uranium enrichment plant, which would take years. Primary reactors could produce weapons-grade plutonium, in addition to electricity. Without further ado, the committee settled on primary reactors.
No, the French government had not decided to build an atom bomb. At the time of these meetings, in 1951, no head of government had seriously considered this possibility.18 But the CEA steering committee members, as the guardians of French nuclear interests, felt that stockpiling plutonium certainly could not hurt. Ostensibly, this plutonium was des tined for breeder reactors in the distant future, but almost everyone on the committee had its military potential in mind. Without specifying the end use, they set a production goal of 15 kilos of plutonium within five years.19
Having made this decision, the committee next had to pick a moderator for its reactor. The choice was between graphite and heavy water, and before continuing, I must digress briefly in order to explain some basics of a fission reactor.
Natural uranium, which France had, contains two isotopes of uranium: U238, and U235. Fission occurs when a U235 atom absorbs a neutron, causing the lighter uranium atom to split and liberating a great deal of energy as well as some more neutrons. Some of these neutrons will be absorbed by more U235 atoms, causing more fission; scientists and engineers at the time understood that with enough uranium piled up—what is known as critical mass—this fission reaction will be self-sustaining. Other neutrons, absorbed by U238 atoms, will not cause fission. Rather, on absorbing a neutron, a U238 atom becomes U239—which eventually changes into Pu239, or weapons-grade plutonium.
The committee members knew that in order for a U235 atom to absorb a neutron, that neutron must be traveling at a speed lower than that at which it is released. Therefore, a moderator was required to slow down the neutrons; further, the ideal moderator would not absorb any neutrons. Finally, in order to extract the heat from the reactor core, a coolant was needed.
By the time the steering committee met in September 1951, the CEA had already built heavy-water experimental reactors.20Physicists preferred heavy water as a moderator because it absorbed fewer neutrons. But heavy water could only be manufactured by electrolysis, which itself required electricity.21 Further, argued the engineers on the committee, a heavy-water manufacturing plant seemed complicated and expensive to build, whereas France already manufactured graphite. Such were the official reasons given for choosing graphite over heavy water as a moderator.
An additional reason, however, was suggested by an engineer who worked on the early gas-graphite designs. Many of those who had worked with the heavy-water research reactors were Communists. Some but not all of these people had been dismissed along with Joliot in 1950. Since the plutonium produced by the first industrial-scale reactors might go into a future French bomb, certain committee members wanted an easy way of excluding Communist scientists and technicians from the new projects. Not picking the technology in which they had experience greatly facilitated this task.22
The CEA steering committee thus settled on a five-year plan for 1952–57 that committed the CEA to building two reactors, powered by natural uranium and moderated by graphite. The plan also included a factory to extract plutonium from the spent uranium fuel which would emerge from the reactors. Pleased with these goals, Gaillard easily convinced his fellow parliamentary deputies to approve the plan. Because it conferred prestige and glory, he argued, France needed nuclear energy. He emphasized the nation’s weakness in energy re sources and noted that expanding the nuclear program meant developing France’s industrial base and ensuring its future energy supply. Without specifying that the reactors could produce weapons-grade plutonium, he opined that France should not publicly renounce the right to build a bomb when countries on both sides of the Iron Curtain had such weapons programs. Presumably because they trusted the experts who had conceived the plan, the other deputies did not question Gaillard on any of the details. With remarkably little debate, they voted a budget of 37.7 million francs to the CEA in July 1952.23 Having funded the plan, Parliament left its implementation to the scientists and grands corps engineers who headed the CEA: after all, most of them had been explicitly trained to serve the state and should be trusted to do so. Parliament had other, more pressing political problems to resolve.
The CEA committee members had chosen the gas-graphite design knowing that it could yield weapons-grade plutonium. But, it was the agency’s new administrator general, Pierre Guillaumat, appointed by the committee in November 1951, who ensured that the reactors did produce this plutonium. Guillaumat had graduated from the Ecole Polytechnique and belonged to the Corps des Mines; he was also a longtime friend and ally of de Gaulle. He aggressively pushed the plutonium production program, while successive heads of government continued to proclaim France’s sole interest in the peaceful atom, at a time when debates within ministerial circles over this position had barely begun.
Guillaumat created the Direction Industrielle to coordinate the construction projects, placing Pierre Taranger, another Polytechnique graduate, at its head. Guillaumat and Taranger made it clear to their top engineers that they had to build a plutonium-production facility as quickly as possible. In less than five years, both the first reactor, G1, and the plutonium extraction factory were operating at Marcoule. Studies for a second, larger reactor—G2—were under way, and Guillaumat had even negotiated an agreement with the Ministry of Defense to build a third plutonium-producing reactor, thereby more than doubling the CEA’s funding.24
Parliament had approved the Gaillard plan in part because it supposedly represented the first step toward a more extensive nuclear energy program. Yet no one even mentioned extracting electricity from G1 until its design was almost finalized. Then Pierre Ailleret, the head of EDF’s research division and a CEA committee member since 1950, suggested appending a 5-megawatt plant to G1.25 Small frictions had already arisen over which institution would provide France with nuclear energy: EDF, the country’s official electricity supplier, or the CEA, the official guardian of all things nuclear. For Ailleret, Gl provided the perfect opportunity to involve an EDF team in the nuclear adventure. The CEA agreed, stressing however that the project should not interfere with plutonium production. With this same caveat, the CEA also agreed to let EDF build a 25-megawatt plant for G2.26
The unstable political climate of the early to mid-1950s, plus government indecision about nuclear policy, thus gave CEA leaders a free hand in shaping the French program.27 By deciding what kind of reactor to build, they, not the government, made French atomic policy. They could not go so far as to openly pursue the military nuclear option—nor did all of them want to. But they could take steps in that direction.
That is but the beginning of the story. Given the inherent ambiguity of this design, we must now seek to understand how engineers and managers in the CEA and in EDF played on that ambiguity in order to further their political and industrial goals. Comparing the contracting process, the design, and the rhetoric surrounding two early gas-graphite reactors—G2 and EDF1—reveals how the reactors became the policy-making tools of engineers.
2.3 Putting Together a Reactor Project, CEA-Style
The method by which Taranger and Guillaumat chose the companies that would design individual components for G2 marked the beginning, in France, of a new kind of industrial policy: the “policy of champions.”28 Both men felt that the best way to convince private industry to participate in a venture that would not yield immediate profits was to handpick the most technologically “advanced” company to design each component, regardless of cost. Such a policy, they argued, meant that French companies would not waste time or resources competing against each other. Further, it would provide a nurturing environment in which industry could develop new technologies. French industry would acquire valuable know-how, which it could use in the future to export technology. Guillaumat and Taranger maintained that this policy would thus enhance France’s industrial base in the short term and its economy in the long term.
Under Taranger’s guidance, the CEA’s Direction Industrielle grouped the chosen companies into a consortium and placed the Société Alsacienne des Constructions Mécaniques (SACM), itself a conglomerate of electrical and mechanical engineering companies, at the head. The CEA signed a contract with the SACM, which subcontracted to the other companies and coordinated the overall design and construction process of G2. The design process was a cooperative effort: after CEA engineers defined the function of a reactor component, industrialists would propose an initial design, which would then be discussed in a series of meetings. Two sorts of meetings occurred: meetings between a CEA team and a single company to talk about a specific component, and large monthly meetings grouping together representatives of all the CEA teams, all the companies, and EDF when relevant.29 The reactor had to be built quickly,30 and this decision-making process had the short-term advantage of producing solutions that industry was capable of building. Left to their own devices, said an EDF engineer present at these meetings, CEA teams would have envisioned complex solutions beyond the means of French industry, and the deadline would never have been met Even so, he added, the solutions chosen were often costly and cumbersome.31
Cost was not a consideration for the CEA, however, and for G2, EDF had little to say in the matter. From the beginning, CEA engineers made it clear that EDF had to play a subordinate role. It had also signed the main contract with the SACM, but the “energy recuperation installation,” as EDF’s part was called, was considered an auxiliary device to the reactor. So while the EDF engineers sat in on the monthly meetings with industry, they were not expected to voice their concerns over the design of their installation. Industry had to give the CEA contracts priority over those signed with EDF.32 Furthermore, EDF engineers did not always know about design changes that had a direct impact on their work.33 As we shall see, this meant that no part of the reactor—as opposed to the generating unit—was designed to optimize electricity production.
The “nuclear” part of the reactor, then, took priority over the “classical” part.34 The “best” companies were chosen to build the trickiest, “most nuclear” parts, with no regard for cost. Contracts signed for such parts were contracts of principle, in which the company agreed to build a device that would perform certain functions, but the specifications were not fixed ahead of time. In contrast, the “less nuclear” parts of the reactor, such as the energy recuperation installation or the prestressed concrete vessel, were covered by contracts detailing both specifications and cost.35
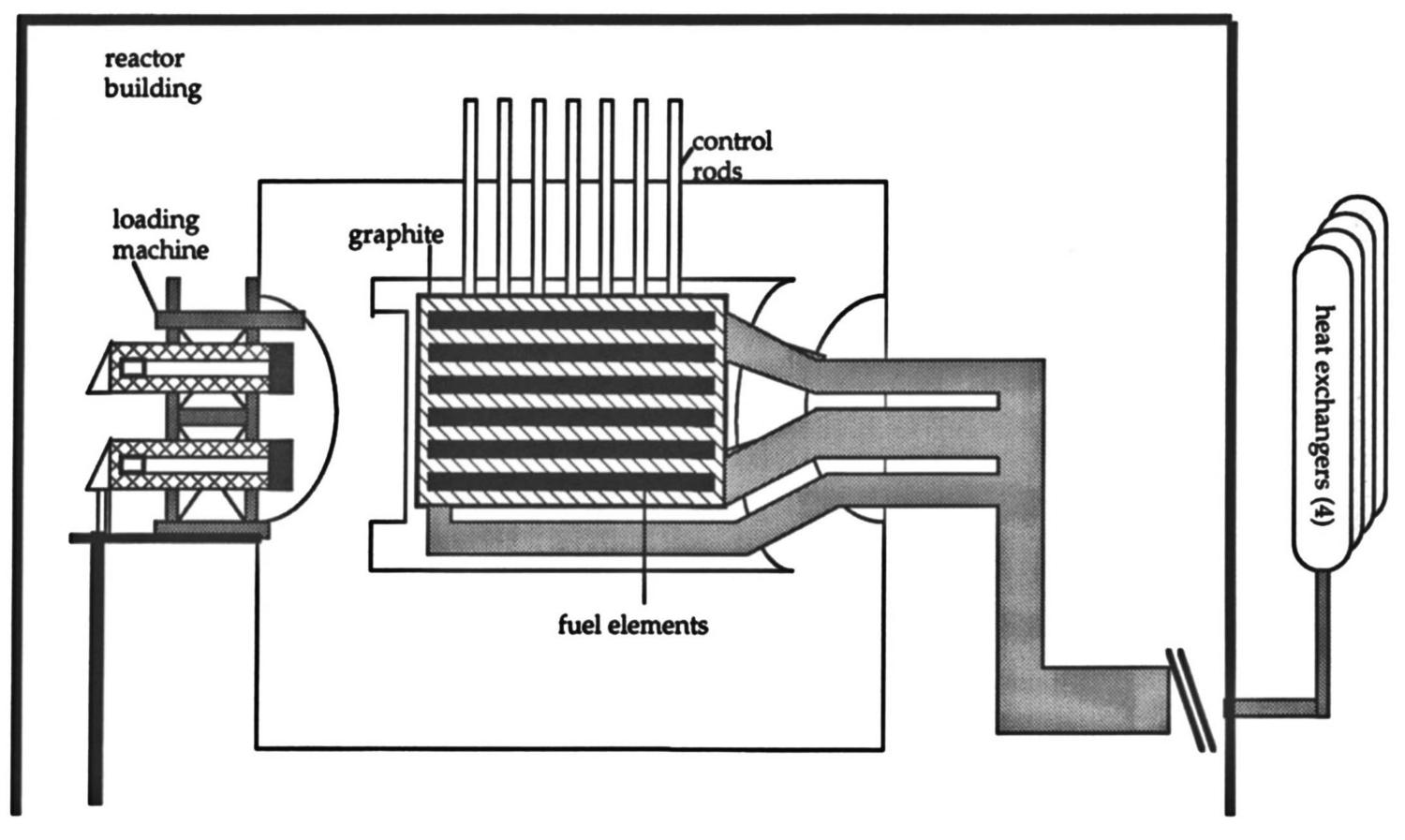
Fig. 2.1: The CEA’s G2 reactor at Marcoule. Schematic diagram adapted from Bulletin d’informations scientifiques et techniques du CEA (1958). Drawing not to scale.
So, what sort of reactor emerged from all this? Figure 2.1 depicts G2. Most of the reactor was housed in a large building designed to protect its contents from the vagaries of the weather (like many of their Soviet counterparts, the Marcoule reactors did not have containment buildings). The core, contained in the large cylinder, was made up of a stack of graphite bars piled in horizontal layers. Distributed through this pile were 1,200 channels, into which the uranium fuel was loaded. The uranium came in small cylindrical rods hermetically encased in aluminum cans. Each channel could contain up to twenty-eight of these uranium rods, or fuel slugs. When enough slugs were loaded into the reactor, it “went critical,” setting off a self-sustaining fission reaction. The fission taking place inside the slugs liberated a great deal of heat, which the cans absorbed. Carbon dioxide gas, entering the channels through openings on the back face, flowed around the slugs and cooled the reactor by absorbing this heat. On leaving the core, the coolant traveled to the “energy recuperation installation” where the heat was converted into electricity.
Even this quick overview of G2 reveals how the electricity-generation function of the reactor was relegated to secondary status. The energy recuperation installation stood outside the building that housed the reactor, both physically and symbolically removed from the fission reaction. In order to show in greater detail how the political agenda of plutonium production took precedence over the political and industrial agenda of electricity production, I will focus on two aspects of G2’s design: the loading and unloading of fuel in the reactor, and the energy recuperation installation itself.
For the CEA, the key point in making weapons-grade plutonium was to obtain as much Pu239 as possible with as few “poisonous” isotopes of plutonium as possible. The Pu239 produced when a U238 atom absorbed a neutron and decayed was not a stable isotope: with time, it changed into Pu240 and Pu241. Among other reasons, these were called “poisonous” because, when present in sufficiently concentrated quantities, they could spontaneously fission.36 A bomb containing too large a proportion of these isotopes might, therefore, explode unpredictably. The CEA team working on Marcoule’s plutonium extraction factory had already settled on a chemically based process to separate the plutonium from the spent uranium fuel, a process which did not distinguish between different isotopes of plutonium. The G2 teams had little knowledge and a severe time constraint to work with; under these conditions, the only solution they could devise that would minimize the poison involved removing the fuel slugs before too much Pu240 or Pu241 appeared. The shorter the time that each uranium slug was irradiated—that is, allowed to undergo fission—the less poison was produced. CEA engineers calculated that at the optimal irradiation for producing the right balance of isotopes, any given fuel slug should not stay in the reactor longer than 250 days.37 In terms of electricity production, this short irradiation period represented extremely inefficient use of fuel: ideally, the slugs should remain in the reactor until the uranium was all burned up for maximum heat yield.
These considerations led CEA engineers to impose a technopolitical constraint on the SACM, the company in charge of designing and building this system. With twenty-eight fuel slugs in each of 1,200 channels, stopping the reactor, then unloading and reloading the core channel-by-channel and restarting the reactor every 250 days would have wasted far too much time.38 And saving time was crucial to the CEA, both technologically, to avoid getting poisonous isotopes of plutonium, and politically, since they wanted the maximum amount of weapons-grade plutonium as quickly as possible. CEA engineers hence asked for a loading system that could function while the reactor was operating.39
SACM engineers chose a costly and cumbersome solution, but it fulfilled the CEA requirements perfectly. A cement block containing tubular holes was built flush against the northern face of the cylindrical vessel. This block contained one tube for each channel. On the far left-hand side the tube connected with the loading device, placed on a traveling crane built on a platform adjacent to this block. The device itself consisted of two lock chambers side by side. By maneuvering the crane up and down and from side to side along the cement block, an operator sitting on top of the crane could couple these lock chambers with any of the channels of the core. Because the operation took place while the reactor was on line, these chambers were constantly exposed to radioactivity. They were therefore encased in 56 tons of metal and concrete.40
The lock chamber linked up to a storage chamber containing the new fuel slugs. New fuel slugs would be loaded onto an elevator and brought up to the storage chamber. The lock chamber, moving back and forth on a track, would pick up the slugs, bring them over to the channel, and a mechanical arm would reach into the tube and undo the plug. The new slugs would be loaded into the channel, pushing the irradiated slugs out. Because this entire procedure took place while the reactor was under pressure, a complex system of locks and sensors had to guarantee a perfectly hermetic seal every time the device coupled with the storage chamber or a channel.41
Fuel loading was by no means the only aspect of G2’s design shaped by its plutonium-production goal. Another example can be found in the CO2 cooling circuit and the energy recuperation installation itself. In essence, this installation contained four heat exchangers, one turbogenerator, and auxiliary equipment. The hot CO2 gas exited the reactor core into the heat exchangers, where it cooled by transferring its heat to water. In the process, the water turned into steam and, after passing through a series of pressure stages, the hot steam would arrive in the turbogenerator, where its heat would be converted into electricity.
Had the main purpose of the reactor been electricity production, EDF engineers would have calculated the pressure, temperature, and flow of C02 that would have yielded the most efficient energy retrieval. G2’s plutonium priority, however, imposed severe constraints on this energy recuperation cycle. First, the reactor had to operate continuously to avoid thermal shock to the fuel slugs. Second, because they had little interest in energy efficiency, CEA engineers had not designed the aluminum cans surrounding the fuel slugs to withstand high temperatures. These two constraints led the CEA to determine specific values for the pressure and temperature of the CO2—values which did not correspond to those for optimal electricity production.42 A third, more significant constraint was that the CEA wanted to operate the reactor at maximum power all the time. Maximum power meant more decays per second of U238 into Pu239. Combined with the rapid unloading of the fuel slugs, this in turn meant that a maximum quantity of Pu239 could appear and be removed before too much of it decayed into poisonous isotopes. Running the reactor continuously at maximum power, however, could not be handled by the electrical network to which the heat generator was hooked up; because of variations in energy consumption, the network could not always absorb all that energy. So all these constraints forced EDF engineers to add a “desuperheater” to the circuit, placed just before the steam generator, to absorb excess heat. Furthermore, the fourth heat exchanger existed only as a safeguard in case of breakdown; in fact, three exchangers would have sufficed to run the reactor and the plant. Finally, in order to run the reactor at maximum power and low temperature, the CO2 had to be blown through the core at a very high rate. This did not favor energy efficiency, first because the high rate required more electricity to power the blower, and second because the exiting CO2 was at a lower temperature, and therefore contained less energy.43
CEA engineers thus translated Guillaumat’s enthusiasm for a French atomic bomb into a reactor design whose ideal function was producing weapons-grade plutonium. The story just told about G2 could easily be that of the other two Marcoule reactors, G1 and G3. While the French government waffled over whether or not to build a bomb, CEA engineers took the crucial first step toward that bomb. They had almost finished building G2 in April 1958, when Felix Gaillard, by that time prime minister, signed the order to have a bomb ready in early 1960. Without these Marcoule reactors, France never could have exploded its first bomb on schedule.
To the engineers and technicians at Marcoule, the military aspect of their work was no secret. A sense of excitement and urgency pervaded the offices in which engineers struggled over design problems and the construction sites where the huge reactors took shape. They were creating a brand new technology, of singular importance to their nation. Although they had indirect knowledge of some American, British, and Canadian nuclear work, they apparently did not have access to many of the technical solutions worked out by Anglo-Saxon researchers.44 They thus relied on their limited experience with experimental reactors and their own ingenuity. Sometimes they favored solutions because they had heard that the British were working on something similar. More often, as explained above, they favored ideas that appeared to provide the quickest, if not always the most elegant, route to completion—and frequently they had no idea whether or not a device would work until it had been built and attached to the reactor. The uncertainty that thus dominated their work created what many later referred to as a “pioneering atmosphere” on the job, and it was this atmosphere and its accompanying excitement that saw them through the sixty- or seventy-hour workweeks that prevailed throughout the project. When the first French atomic bomb, loaded with plutonium produced at Marcoule, exploded on February 13, 1960, one engineer said that he and his colleagues were so proud of their country and the part that they had played in this achievement that they “shed tears of joy.”45
The characterization of Marcoule as a French achievement, piloted by the CEA and implemented by French industry, pervaded the science and engineering press.46 Some descriptions of Marcoule even referred to great French engineering achievements of the past. For example, in the introduction to a special journal edition devoted exclusively to G2 and G3, the CEA’s high commissioner Francis Perrin wrote: “[The CEA] deemed it necessary to associate French industry with these great achievements which prepare the way for the development of the industrial use of nuclear energy. French industry answered this appeal, despite deadline and supply constraints that were often severe. … Above all, the result of this collaboration is apparent through the two massive buildings that dominate, by their 50 meters, the banks of the Rhône. [They are] a modern replica of the ancient wall of Orange that faces them.”47 And in an article for a mining engineering journal, the director of the Marcoule site gave his readers an idea of the scale of the reactors: “The Arc de Triomphe of the Étoile would easily fit in the vast metallic structure that shelters … G2.”48
Before Gaillard signed the bomb order in April 1958, however, the fact that the plutonium that would be produced at Marcoule was destined for use in a bomb remained secret from those not intimately involved with the nuclear program. Thus, G2 both constituted French nuclear military policy and represented that policy’s ambiguity. Ostensibly, the plutonium produced therein was destined for future experiments and reactors. G2 itself could in all legitimacy be presented as a prototype for a power-producing reactor—what other reason, after all, would EDF have for being involved with the project? That G2 did in fact produce a modicum of electricity (25 megawatts on a good day) enabled engineers and managers to speak of G2 as a successful prototype worthy of the tremendous investments made in the nuclear program. A 1957 article in a French civil engineering journal said:
The first stage of the plan called for the construction of two nuclear reactors (Gland G2) that were supposed only to produce plutonium destined to fuel the secondary reactors of the future. … But during the study, we were led to envisage using the heat released by these reactors to produce electric energy.
Currently, the predicted total investment amounts to 60 billion francs. This financial effort is justified by the necessity to develop, on the industrial scale, the production of electrical energy of nuclear origin, due to the insufficiency of European resources in fossil fuel. The role of Marcoule is essentially to allow this development, to train teams of operators at different levels, and to promote technical and industrial progress in this field.
Investments should thus not be measured against the power of the installations, but against the development potential that they bring. In fact, the [amount of these investments] is quite in proportion to the increase in our energy needs.49
This was by no means the only such article written in the French engineering press. Seemingly, CEA personnel felt a need to justify their privileged financial status to their fellow engineers. In this way, the Marcoule reactors were the perfect instrument of French nuclear policy; they had primed the path to the French bomb, but until this path became public, they could easily be justified in terms of electricity generation.
We can see a striking example of the role played by the ambiguity of the Marcoule designs in French nuclear policy by briefly examining the first ministerial level discussions on the French bomb. These occurred in late 1954 in a series of meetings presided over by Pierre Mendès-France, then head of government. Those present included Guillaumat and Perrin of the CEA, as well as the minister of finance, the minister of national defense, the secretary of state for research, and various ministerial cabinet members. Guillaumat, the national defense minister, and others in favor of building a French bomb tried to push Mendès-France to make an official decision to that effect, arguing among other things that a bomb effort would have advantageous fallout for the civilian sector.50 In the words of Mendès-France:
I remember asking which part of the research under way was of economic interest, and which was only of military interest. They retired to a comer of my office to discuss matters in a low voice, and several moments later, they came back and told me, “for another three years, we won’t be able to distinguish the military from the civilian; only after three years will we reach a branching point when we can say: this is purely military, and that holds a purely economic interest.” Under those conditions, I said, there’s no problem: we must continue to do research. … There was no question of amputating the positive aspects of such research work from the French economy.51
Thus Mendès-France, who until that moment had been one of the most decisive and forceful leaders of the Fourth Republic,52 chose not to decide. His government only lasted two more months, so he never reached that fateful “branching point.”
Successive governments also flirted with making a firm decision. Until the Gaillard government, though, they all shied away from this task.53 Thus, the ambiguity of the Marcoule design stood Guillaumat and others in favor of the French bomb in good stead. Depending on the political climate, they could bill the Marcoule reactors, which were the centerpiece of the CEA program, as purely civilian, purely military, or somewhere in between. Meanwhile, Guillaumat’s engineers had inscribed a military agenda into the reactors, enabling France to stroll discreetly toward the bomb.
2.4 Building a Reactor, EDF-Style
In early 1955, shortly after the start of the G2 project, top engineers in EDF and the CEA drafted a long-term gas-graphite reactor program. EDF1, a 60-megawatt electricity-generating reactor, was the first step in this plan. A succession of increasingly powerful reactors would follow, which by 1965 were to produce a total of 800 electric megawatts. The engineers submitted their plan to the PEON commission,54 recently formed as a government advisory council on matters of nuclear energy development. Members of PEON included high-level engineers and managers from both institutions; not surprisingly, the commission approved the plan.55 In July 1955, engineers began designing EDF1.
Despite the fact that the Marcoule reactors did not produce energy in the way that EDF wanted them to, the CEA’s efforts there did benefit the utility both technologically and politically. It had, after all, been able to experiment a little with nuclear energy production. Furthermore, it had neither the time, money, or expertise to launch an independent nuclear program. Politically, EDF’s participation in the Marcoule projects had buttressed the CEA’s claims that the Marcoule reactors were prototypes for power reactors. Conversely, Marcoule’s success strengthened EDF’s case for funding its own power reactors. Clearly, the two agencies collaborated in large measure because they needed each other.
In the course of working out the parameters of this collaboration, however, each agency was also eager to establish its role in defining the future not only of the nuclear program but also of French industrial development. In theory, the terms of cooperation were clear. CEA teams would design the “nuclear” parts of the reactor, and EDF teams would design the “classical” parts, but ultimately EDF headed the project and would thus make most of the final decisions. In practice, though, the EDF1 project was fraught with tension between engineers in the two institutions. This tension centered around two issues: the role of private industry in the project, and the actual design of the reactor. Conflicts did not emerge because the CEA did not want to build an electricity-producing reactor. Part of the CEA’s mission was to develop nuclear technology in any form. But CEA engineers wanted to build these reactors their way. Further, they wanted to preserve the dual nature of the gas-graphite design, thinking that they might eventually get some plutonium out of EDF reactors, just as EDF had gotten some electricity out of Marcoule. Engineers in each institution considered their particular expertise as the key to designing EDF1: CEA engineers held that their intimacy with nuclear knowledge gave them the edge, while EDF engineers maintained that their experience with conventional power plants gave them the upper hand.
Finally, more was at stake than the EDF1 project alone. It was still far from clear that the nuclear program would receive long-term support. Precisely what would receive support (a military program, a civilian program, or both)—and to what extent—was also uncertain, particularly before 1958. Furthermore, project participants expected that the set of working methods and expertise that prevailed in the EDF1 endeavor would surely dominate, or at least influence, future reactor projects; they therefore felt that they were conceiving not just one but a whole series of French nuclear reactors.56 In the 1960s, once the nuclear bomb program stood on firm ground and EDF had clearly established its authority over nuclear energy production, tensions between the two agencies lessened considerably.57 But in the mid- to late 1950s, each institution was fighting for its future and the future of France.
The point of examining the EDF1 project, however, is not to find out who won this series of conflicts: almost invariably EDF did, because the protocols governing the collaboration did give EDF the final power of decision over its own reactors. Rather, the point is to understand how EDF engineers inscribed their political, economic, and technological agendas into the project and made EDF1 into their own policy-making tool. The conflicts, then, serve as additional proof that each institution really was doing more than merely designing a reactor: each was trying to impose a way of creating technology and a route for French nuclear development.
Tensions between the two institutions first became manifest in the organization of the project. As in the case of G2, Guillaumat and Taranger wanted private industry to coordinate the design and construction of EDF1. The nuclear team at EDF, however, believed that it should have the role of project coordinator58—the function that the SACM had fulfilled for G2. Team members espoused the anticapitalist sentiment that had originally led to the nationalization of the electric utility. By building EDF1, they were providing a public service. The best way to do this was to optimize the cost and efficiency of the reactor.59 Ailleret, who by then had risen to the post of managing director of the utility, argued that EDF, not private industry, should conduct the optimization studies “in order to be sure that we are not influenced by the industrialist’s tendency to develop certain types of materials rather than others.”60 Another team leader commented disparagingly, “Guillaumat and Taranger [were] oil men, who dreamed only of private industry.”61 Furthermore, it seemed appropriate that EDF should coordinate the overall design and building as well. The best way to keep costs down, EDF engineers argued, was to divide the reactor into parts and launch requests for bids for each part. EDF would thus have greater control over both the knowledge needed to build the reactor and the cost of the project. To top it all, this method of working was “politically correct”: in the ironic words of a high-level EDF manager, “The pure and white EDF, a nationalized company, would acquire the know-how while leaving the builders, the capitalist companies, with the banal task of supplier.”62
Overriding the objections of Guillaumat and Taranger, the EDF team decided to proceed in this manner.63 The first step now was to draft a preliminary design project. Jean-Pierre Roux, the head of EDF’s design team, had asked the CEA to do so in July 1955. EDF engineers found this proposal, heavily based on G2’s design, unacceptable: they intended to generate electricity “optimally,” something G2 did not do.64
The EDF team sought to change practically everything in the CEA’s preliminary proposal.65 In order to optimize the reactor for electricity generation, they wanted to control the definition of almost all the components and parameters, including components such as the uranium-graphite pile and the devices for loading and unloading the fuel, as well as parameters such as the pressure of the CO2 cooling gas and the operating power of the reactor.66
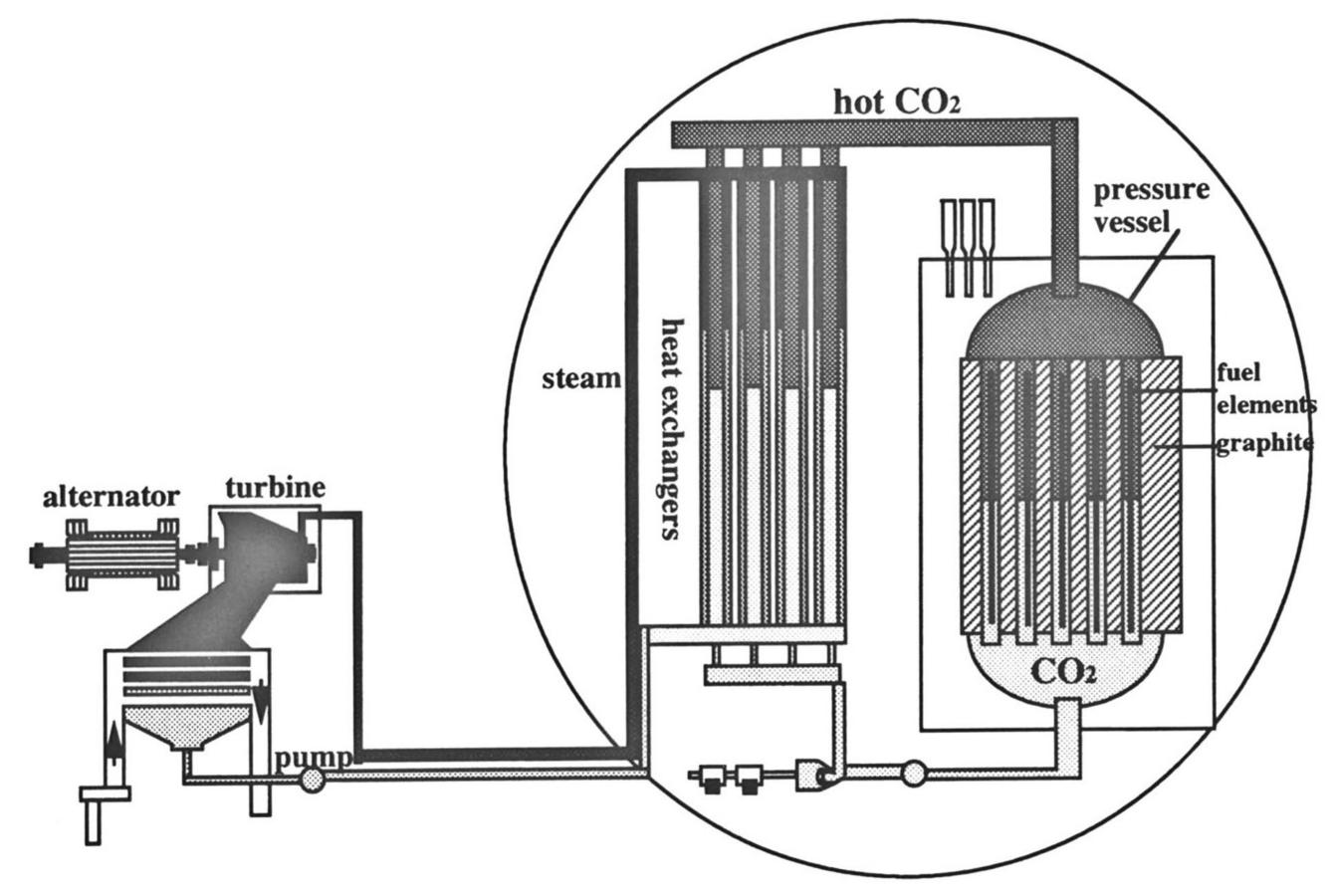
Fig. 2.2: The EDF1 reactor at Chinon. Schematic diagram based on EDF’s Rapport de sûreté Chinon A1 (1980). Drawing not to scale.
The finished design of EDF1, shown schematically in Figure 2.2, looked quite different from that of G2. The most noticeable and perhaps the most symbolic change was that the heat exchangers were right next to the pressure vessel that contained the core, rather than many meters of energy-losing pipes away, and inside the reactor building (which was a spherical containment structure, unlike G2’s building) rather than outside. The reactor still ran on natural uranium, encased in fuel slugs similar to those of G2; it was still moderated by graphite and cooled by CO2. Just about everything else, however, had been modified.
The EDF team insisted on changing the operating pressure of the reactor and the pressure vessel containing the core. The CEA team had suggested a prestressed concrete vessel like the one at Marcoule. It argued that, in addition to being a tested technique, prestressed concrete was a domain in which France had outdistanced other nations. CEA officials felt a responsibility to encourage French industry to reinforce its areas of excellence.67 But EDF found this vessel too expensive. The prestressed concrete could not withstand the temperatures at which EDF planned to operate the reactor and would require a special cooling circuit. This would increase overall operating costs and lower the efficiency of the reactor: the blowers needed to pump the CO2 through the special circuit would use up 10 percent of the electricity generated by the reactor.68 Instead, EDF engineers chose an all-steel vessel, cylindrical in shape, capped by a steel hemisphere on either end.69 A steel vessel could withstand higher temperatures and pressures. In addition, the fact that the United States and Great Britain had built steel vessels for their reactors gave EDF project engineers confidence that they too could build a working steel vessel for their reactor. They cared less about promoting technology uniquely or distinctly French than about using the cheapest, most reliable techniques.70
Early on, EDF engineers decided that EDF1 should function at a higher pressure than G2, with twenty-five bars instead of fifteen. Lower pressure reactors were easier and faster to build, and speed had been of prime political importance to CEA engineers in the G2 project. But a lower operating pressure meant that a higher flow of CO2 was needed to extract the heat, which required more powerful blowers, thereby lowering the efficiency of the reactor.71 EDF engineers had also decided, from the beginning of the project, that the loading and unloading of the fuel would take place while the reactor was stopped. Unlike the CEA, EDF wanted to bum up the fuel slugs as much as possible in order to extract the maximum amount of heat. It had no use for a device that could move slugs in and out of the reactor at a high pace. Building a loading device that could work only with a stopped reactor limited the amount of weapons-grade plutonium that the CEA could demand from EDF1.72
Having decided on this loading principle, the EDF team then decided to orient the channels containing the slugs vertically, rather than horizontally as in G2. In a vertical configuration, the CO2 could be pumped in at the bottom. It would thus follow the natural convection of heat, reaching the hotter parts of the reactor as it rose. This meant that less pumping power was required for the CO2 and made the overall design safer in case of blower failure. A vertical pile also led to fewer openings in the pressure vessel, thereby making it easier to ensure that the core was hermetically sealed. It also meant that the reactor could be loaded and unloaded from the bottom. Bottom loading involved using “a single loading arm capable of reaching all the channels and requiring only one opening in the shell, although clearly a large one.”73 EDF engineers found this system much simpler and cheaper than the G2 design,74 which had, as we saw, separate openings in the vessel for each channel and a huge, heavy machine designed to have access to every channel.
EDF engineers thus advocated a design they felt would make most efficient use of both fuel slugs and investments and that would be as simple as possible so as to provide a good basis for future reactors.75 Both through the design itself and through the industrial contracting process, EDF engineers sought to redefine what a reactor was, how it should be built, and what it should be used for. By modifying pressure or temperature, EDF engineers had changed the performance and the capabilities of the reactor. They wanted to ensure that in future collaborative efforts, the CEA would have to work with such new parameters.
EDF engineers found that the process of designing a power reactor involved a great deal of guesswork and intuition. In the mid-1950s, they had no more access to foreign technology than did their CEA colleagues; at that time, furthermore, no nation had a functioning power reactor. They thus chose technical solutions that they thought would further their political, economic, or industrial goals. Often, they assumed solutions had to be fundamentally different from those advocated by the CEA. By the time they began designing EDF4 in the mid-1960s, they had revised several such solutions, finding ways, for example, to make prestressed concrete vessels and continuous fuel loading suit their purposes. What mattered in the mid-1950s, though, was that those within the utility and in the relevant ministries believed that EDF1 engineers had designed the project that best fulfilled the utility’s goals.
EDF engineers’ view of their work shows in the way they promoted their achievements to other French engineers—inside as well as outside their institution, for not everyone at EDF believed that nuclear energy could ever compete with conventional power plants.76 Some of their prose paralleled that of CEA engineers, explaining that “anguishing” shortages in energy resources justified the huge “financial sacrifices” made for the nuclear program, sacrifices which, in any event, would soon pay off, since nuclear energy increasingly seemed like a “providential solution.”77 At the same time, though, EDF engineers tended to discuss ways to improve reactor technology more readily and more specifically. In particular, some looked to the day when they would no longer have to use natural uranium, a choice in which they had played no part: “The inferiority of natural uranium piles is less economic than it is energetic. Later, when we move to another kind of reactor that allows us to use enriched fuel, it will be less to lower the cost of the kWh than to reduce the specific consumption of fuel and increase, in considerable proportions, the amount of energy that can be drawn from natural reserves.”78
Indeed, EDF engineers had reason to be preoccupied with the overall “efficiency”—both energetic and economic—of their electricity-generating technologies. In order to get France’s energy sector back on its feet, EDF had focused, after the war, on building as many conventional power plants as possible, as quickly as possible. The resulting hydroelectric program had therefore paid less attention to cost than it had to speed and reliability. In the face of sharp criticism in the early to mid-1950s, EDF had adopted an institution-wide policy of rentabilité, best translated here as “economic viability,” which coincided with the priorities of the Second Plan elaborated by the nationwide Planning Commission.79 Engineers hence had to show that their designs would not lose money and would make efficient use of fuel. Already, the engineers who had built the hydroelectric plants were fighting with those in charge of thermal plants over whose work best fulfilled these requirements.80 EDF’s nuclear team therefore aimed its arguments about the benefits of nuclear energy as much at other, nonnuclear EDF engineers as at the world outside EDF.
It is also significant that EDF engineers compared their achievements with those of other nations, especially England. Jean-Pierre Roux compared EDFl with the Calder Hall reactor and concluded, “After this rapid overview of the principal characteristics, it appears that this French project holds up under comparison with the English projects.”81 This became even more true, he said, when one considered that the British took five to six years between reactors, whereas the French were only taking two.
Finally, EDF engineers waxed eloquent on the benefits of nuclear energy to emphasize that by building nuclear reactors, they were fulfilling their mission of public service to the French state and the French people:
The path taken in giant steps during the past few years in the four large atomic countries, and especially in France, allows the highest hopes.
It is not chimerical to think that the moment of massive realizations approaches rapidly.
Placed at the disposal of all, in the workshop and in the home, nuclear energy will allow economic and social progress to continue everywhere in the world, and in the European community in particular.
France must reap the moral and material benefits that she has the right to expect from a technology so often fecundated by her scientists and already so widely developed by her engineers.82
2.5 Conclusion
We have seen here two gas-graphite reactors, two ways of organizing technological work, and two ways of promoting that work. There existed no single best way to build these reactors; they were not the inevitable results of some progressive logic inherent in the technology itself. Rather, each embodied a different set of political, economic, industrial, and technological agendas. The reactors were thus as much political artifacts as they were technological artifacts.
The story further reveals the importance of examining technology when discussing national policy-making. French military nuclear policy in the 1950s was not made by government officials carefully analyzing their nation’s place in the postwar world and firmly deciding to build a bomb. In the political chaos of the Fourth Republic, officials worried primarily about their own political survival; in the 1950s, such concerns precluded any deep consideration of nuclear policy. Heads of state, ministers, and elected officials were more than happy to let engineers and managers in state-owned agencies do most of the work toward formulating a nuclear policy for France. And so, in the absence of a firm decision about a French bomb, the Marcoule reactors were France’s nuclear military policy, containing both the ambiguities and ambivalences of Fourth Republic governments and the agendas of CEA technocrats. Grands corps engineers such as Guillaumat and Taranger insisted that their personnel inscribe in the Marcoule reactors their conviction that France should build an atomic bomb: thus, technological work became political work.
The Marcoule reactors did not make a French bomb inevitable, but they did, by virtue of the tremendous technological, financial, intellectual, and organizational resources poured into them, constitute one of the most powerful arguments in favor of that bomb. Historians, politicians, and others have debated ad nauseum the question of who “decided” to build the French bomb and when that “decision” took place.83 By delving into reactor technology and examining the interaction of technology and politics, we can see that framing the question in that way makes no sense. In the tumultuous political climate of Fourth Republic France, developing an atomic bomb was a process, not a decision, and it was a process in which technocrats, engineers, and the technology that they produced played a far greater role than did politicians.
CEA technology also shaped France’s nuclear energy policy. The Marcoule projects proved important to EDFl project engineers both technologically and politically. Lack of expertise, time, and money prevented EDF engineers from designing a different kind of reactor. But they were able to transform the gas-graphite design, imbuing it with their own imperatives of electricity production, efficiency, and simplicity. Thus, they too made policy through their technological work. They played on the inherent ambiguity of the gas-graphite design to direct French nuclear policy more firmly toward energy production. They used their technological choices in the EDFl project—and the fact that those choices differed from the CEA’s—to convince other engineers in their institution, as well as politicians who could fund their program, that nuclear energy could present a viable economic alternative to conventional power sources.
In the early to mid-1950s, the two agencies had obtained funding for their programs by making vague allusions to issues of international prestige and long-term industrial and economic development. By the late 1950s, the technological and political work that the CEA and EDF had accomplished together had buttressed these earlier arguments and firmly established the nuclear program as an arena in which issues of great significance to the French nation would be played out. But although they had a common interest in promoting a nuclear program and a shared heritage of public service, engineers and managers in the two institutions had different conceptions of the public interest and specific visions of their role in the future of their nation. So when CEA and EDF engineers sought to define the relationship between private industry and state enterprises that would best promote the nation’s industrial and economic development, they engendered two different definitions. The CEA’s “policy of champions” reflected the Gaullist tendencies of that institution. EDF’s policy of picking the lowest bidder and controlling the overall design stemmed, perhaps ironically, from its left-wing desire to keep economic control of energy production firmly in the hands of a state agency. Each institution’s policy, and resulting technology, embodied a pole of Fourth Republic politics. The nuclear program thus became a testing ground for broader issues in France’s economic and industrial policy.
2.6 Acknowledgments
This work was funded in part by grants from the National Science Foundation, the Mellon Foundation, and the Institute of Electrical and Electronics Engineers. An earlier version of the article was awarded the Levinson Prize by the Society for the History of Technology and was presented at the 1991 SHOT meeting in Madison, Wisconsin, and several other locations. The author thanks Thomas P. Hughes, Robert Frost, Nina Lerman, Eric Schatzberg, David Shearer, and a Technology and Culture reviewer for their comments on previous drafts of this article.
2.7 References
Asselain, Jean-Ciaude (1984). De 1919 à la fin des années 1970. In: Histoire économique de la France du XVIIIe siècle à nos jours. Vol. 2. Paris: Éditions du Seuil.
Bedarida, F. and J.P. Rioux, eds. (1985). Pierre Mend ès France et le mendèsisme: l’exp érience gouvernementale (1954–55) et sa post érité. Paris: Fayard.
Bienvenu, Claude (1956). “Étude préliminaire d’une installation de récupération sur EDF1”. Personal papers.
– (1957a). “Étude des réacteurs énergétiques EDF, projet d’organisation dans le cas d’un réacteur du type uranium naturel-graphite-CO2”. Personal papers. March 8.
– (1957b). “Memo RETN 1”. Personal papers. July 25.
– (1963). “Ailleret’s declaration to the Conseil Économique et Social”. Personal papers. June 27.
Bonin, Hubert (1987). Histoire économique de la IVe République. Paris: Economica.
Brun, Gérard (1985). Technocrates et technocratie en France, 1918–1945. Paris: Albatros.
Buffotot, Patrice (1987). Guy Mollet et la défense: Du socialisme patriotique au socialism atlantique. In: Guy Mollet, un camarade en r épublique. Ed. by Bernard Ménager, Philippe Ratte, Jean-Louis Thiébault, Robert Vandenbussche, and Christian-Marie Wallon-Leducq. Lille: Presses universitaires de Lille, 499–514.
Bulletin d’informations scientifiques et techniques du CEA (1958). Vol. 20. Le Commissariat à l’énergie atomique et aux énergies alternatives (CEA).
Bungener, Martine (1986). L’électricité et les trois premiers plans: Une symbiose réussie. In: De Monnet à Massé: Enjeux politiques et objectifs économiques dans le cadre des quatre premiers Plans. Ed. by Henry Rousso. Paris: Ed. du Centre national de la recherche scientifique, 107–20.
Coutrot, Aline (1985). La politique atomique sous le gouvemement de Mendès France. In: Pierre Mend ès France et le mendèsisme. Ed. by F. Bedarida and J.P. Rioux. Paris: Fayard, 309–16.
de Rouville, M. (1958). Le centre de production de plutonium de Marcoule: Sa place dans la chaine industrielle de l’energie nucléaire. Revue de l’industrie nucl éaire 40: 483–89.
Ertaud, A. and G. Derome (1958). Chargement et déchargement. Bulletin d’informations scientifiques et techniques du CEA 20:69–88.
Frost, Robert L. (1991). Alternating Currents: Nationalized Power in France, 1946–1970. Ithaca, NY: Cornell University Press.
Goldschmidt, Bertrand (1980). Le complexe atomique. Paris: Fayard.
– (1987). Les pionniers de l’atome. Paris: Stock.
Hughes, Thomas P. (1989). American Genesis: A Century of Invention and Technological Enthusiasm, 1870–1970. New York: Viking.
Kieffer, J. (1963). La centrale de Marcoule: Expérience, résultats et enseignements dans le domaine de la production d’électricité. É nergie nucléaire 5(4):250–256.
Kuisel, Richard (1973). Technocrats and Public Economic Policy: From the Third to the Fourth Republic. Journal of European Economic History 2:53–99.
Lamiral, Georges (1988). Chronique de trente ann ées d’é quipement nucléaire à Électricité de France. Paris: Assoc. pour l’Histoire de l’Électricité en France.
Larkin, Maurice (1988). France Since the Popular Front: Government and People, 1936–1986. Oxford: Clarendon Press.
Leo, Kaplan, and Segard (1958). Problems of Fuel Loading and Unloading in Reactor EDF1. Geneva Conference 33(A/CONF.15/P/1201):582–90.
MacKenzie, Donald (1990). Inventing Accuracy: A Historical Sociology of Nuclear Missile Guidance. Cambridge, MA: MIT Press.
Massé, Pierre (1965). Le plan ou l’anti-hasard. Collection Idées 78. Paris: Gallimard.
McGaw, Judith (1987). Most Wonderful Machine: Mechanization and Social Change in Berkshire Paper Making, 1801–1885. Princeton, NJ: Princeton University Press.
Nous avons visité pour vous ... le centre français de production de plutonium à Marcoule (1957). É nergie nucléaire 1(3):141–44.
Papault, R (1957). Le centre de production de plutonium et d’énergie électrique d’origine nucléaire de Marcoule (Gard). Le g énie civil 134:389–98.
Passérieux, P. and R. Scalliet (1958). Installations de récupération d’énergie. Bulletin d’informations scientifiques et techniques du CEA 20:99–114.
Perrin, F. (1958). Avant-propos. Bulletin d’informations scientifiques et techniques du CEA 20.
Peyrefitte, Alain (1976). Le mal fran çais. Paris: Librairie Plon.
Pfaffenberger, Bryan (1990). The Harsh Facts of Hydraulics: Technology and Society in Sri Lanka’s Colonization Schemes. Technology and Culture 31:361–97.
Picard, Jean-Franois, Alain Beltran, and Martine Bungener (1985). Histoires de l’EDF: comment se sont prises les décisions de 1946 à nos jours. Paris: Dunod.
Rapport de sûreté Chinon A1 (1980). Tech. rep. Électricité de France (EDF).
Rioux, Jean-Pierre (1980). L’ardeur et la n écessité. Vol. 1. La France de la Quatrième République. Paris: Éditions du Seuil.
– (1983). L’expansion et l’impuissance, 1952–1958. Vol. 2. La France de la Quatrième République. Paris: Éditions du Seuil.
Rousso, Henry, ed. (1986). De Monnet à Massé: Enjeux politiques et objectifs économiques dans le cadre des quatre premiers Plans. Paris: Édition du Centre national de la recherche scientifique.
Roux, Jean-Pierre (1957). La Centrale Nucléaire EDF1 de Chinon. M émoires de la Société des ingénieurs civils de France 110(4):294–304.
Scheinman, Lawrence (1965). Atomic Energy Policy in France under the Fourth Republic. Princeton, NJ: Princeton University Press.
Simonnot, Philippe (1978). Les nucl éocrates. Grenoble: Presses universitaires de Grenoble.
Smith, Cecil O. (1990). The Longest Run: Public Engineers and Planning in France. American Historical Review 95(3):657–92.
Suleiman, Ezra (1978). Elites in French Society. Princeton, NJ: Princeton University Press.
Teste, Yvan (1957). Les installations de production d’énergie de Marcoule et la Centrale Nucléaire de Chinon. M émoires de la Société des ingénieurs civils de France 110(2).
Thépot, Andre (1985). L’Ing énieur dans la société française. Paris: Les éditions ouvrières.
Thoenig, Jean-Claude (1973). L’è re des technocrates: le cas des ponts et chaussées. Paris: Edition l’Harmattan.
Vallet, Bénédicte M. (1986). The Nuclear Safety Institution in France: Emergence and Development. PhD thesis. New York University.
Weart, Spencer R. (1979). Scientists in Power. Cambridge, MA: Harvard University Press.
Footnotes
Works that have implicitly or explicitly made this point recently include (but are by no means limited to) Hughes (1989); McGaw (1987). I do not wish to imply that mainstream historians have ignored works in the history of technology or that these works have not contributed to other areas of historical scholarship—they have done so most fruitfully. But, studies of technological design have tended to concentrate on deconstructing the processes of invention, development, and diffusion, thereby speaking primarily to audiences already interested in technology. Such analyses have many virtues, but contributing to broader historical debates is not among them.
The minister of industry who pushed through the nationalization of EDF, Marcel Paul, had been appointed by de Gaulle; however, he was also an active member of the Communist labor union, the Confédération Général du Travail, and de Gaulle had appointed him in pan for this reason. Fourth Republic governments were generally coalitions of those parties best represented in the National Assembly, and in 1944–46 the Communists made up the single largest faction in the assembly. De Gaulle thus had to have Communist ministers in his cabinet, something to which he was not averse in this period, as the Communists had been among the most active members of the Resistance.
Gaillard was the Secretaire d’État à la Présidence du Conseil under René Pleven (1951), Edgar Faure (1952), Antoine Pinay (1952), and René Mayer (1953). He had authority over the CEA thanks to the following decrees: August 14, 1951; January 23, 1952 (52-105); March 22, 1952 (52-328); January 10, 1953 (53-10). See Lamiral (1988).
This committee was made up of ten members, haut fonctionnaires scientific or industrial leaders, and presided over by either the prime minister or his representative.
These are “anciens francs.”
In his various books, Bertrand Goldschmidt also asserts that the military goal of plutonium production was at least tacitly understood by everyone. See, e.g., Les pionniers de l’atome (1987) and Le complexe atomique (1980). This assertion was widely confirmed by the many CEA engineers whom I interviewed in 1989–90.
During the course of this research, I interviewed about seventy engineers who worked either for the CEA or EDF in the 1950s and 1960s. For legal and procedural reasons, I cannot provide individual names for specific citations. I can, however, list the names of interviewees used as sources for this article (interview dates are in parentheses): Pierre Bacher (May 11, 1990), Claude Bienvenu (October 27, 1989), Rémy Carle (February 27, 1990), André Grégut (June 18 and 20, 1990), Adrien Mergui (December 18, 1989), Jean-Pierre Roux (December 20, 1989), Boris Saïtcevsky (February 27, 1990), André Teste du Bailler (November 28, 1989), and Pierre Zaleski (December 22, 1989).
The Communist deputies, whose party had recently focused on obtaining signatures for the Stockholm Appeal, a worldwide petition calling for a ban on nuclear weapons, tried to introduce a clause into the plan that would formalize France’s commitment to the peaceful atom. The rest of the assembly interpreted this effort as a piece of Communist propaganda, and the clause was shelved. There was thus no parliamentary discussion of the military implications of the plan. For more on parliamentary action—or inaction—on this issue, see Scheinman (1965, 8).
Ailleret was in fact EDF’s first representative at the CEA. In principle, other members of EDF who should also have been involved in CEA committees were the président du Conseil d’Administration (decree of January 3, 1951); the directeur général or one of his adjuncts (April 19, 1951); two directeurs généraux adjoints (December 12, 1952, and November 18, 1952). See Lamiral (1988).
This is Scheinman’s thesis (1965, 8), but he reaches this conclusion in a political study without examining the meetings in which the gas-graphite decision took place, much less the reactor design itself. He is therefore unable to say much about the tools and tactics used by engineers and managers to shape the nuclear program, or about the implications of their having had such powers.
Virtually all of the CEA personnel I interviewed stressed that solutions had to be chosen and implemented quickly because they were in a hurry to get sufficient quantities of weapons-grade plutonium to make one or more bombs.
For example, in a letter to the CEA’s Direction Industrielle dated March 4, 1958, accompanying a report on the “Installations de récupération d’energie G2-G3” (personal papers of Claude Bienvenu, interviewee), Georges Larniral wrote, “We attract your attention to the following fact: as a result of the latest modifications effected on the fuel load, the Société Rateau put together a study in order to determine the new characteristics of the CO2 in the [energy] recuperators. The results of this study have not been communicated to us” (my translation).
The terms “nuclear” and “classical” were used by CEA and EDF engineers alike throughout the 1950s and 1960s.
More specifically, even-numbered isotopes of uranium and plutonium are not fissionable, and for this reason Pu240 was a “poison.” Plutonium 241 was spontaneously fissionable.
Power is a measure of energy per unit time; in the case of a nuclear reactor, it measures how many decays occur in a given time period. G2 operated at a power of around 200 thermal megawatts (MW) and contained 100 tons of uranium. The optimal irradiation (measured in power multiplied by the number of days in the reactor, divided by the tonnage of uranium) was set at 500 MW days/t. To find the number of days, engineers made the following calculation: 500 MW days/t = [(200 MW)/(100 t)] x N days. So N days = [500 MW days/t] x [100 t/200 MW] = 250 days, the amount of time a slug should remain in the reactor (data obtained from interview).
From the standpoint of today’s reactor technology, one might imagine that EDF would also be interested in loading the core while the reactor was on line in order to avoid losing money in plant downtime. As we shall see in the section on EDF’s reactor, however, the technical and economic considerations that EDF engineers privileged in the mid- to late 1950s led them to prefer loading reactors that were stopped rather than those that were operating.
Interviews (21); Ertaud and Derome (1958, 69–88).
Ertaud and Derome (1958, 69–88).
Ertaud andDerome (1958, 69–88).
According to the CEA personnel interviewed, they did not gain access to the knowledge that the British had accumulated while building gas-graphite reactors until well into the Marcoule projects. Rumors exist that some scientific and technical knowledge was secretly imparted to the French by the British, but even if these are true the amount of knowledge transferred in the mid-1950s is still likely to have been rather small.
Interview.
Papault (1957, 389–398) (my translation). Measuring the worth of a large technological program in terms of its general value to the nation rather than in terms of direct economic returns on investment was an argument with a long tradition among French public engineers. See, e.g., Smith (1990, 683).
Quoted in Simonnot (1978, 228–29) (my translation).
Its full name was the Commission Consultative pour la Production d’Électricité d’Origine Nucléaire; it was created in April 1955.
The first members included Guillaumat, Perrin, and Taranger from the CEA, and R. Gaspard, Ailleret, and R. Guiguet from EDF.
Tension was such that Ailleret insisted on naming the reactor EDFl rather than G4—or, as it was later known (once EDF had firmly established its place in the nuclear program), Chinon Al (information based on interview).
Frost (1991) shows that in the 1960s tensions arose less between the two institutions than within EDF, between engineers involved in the technical design of power plants and financial experts concerned with medium-and long-term economic planning.
This role was officially termed “industrial architect.” The industrial architect coordinated the overall project, fitting the various components of the reactor into a whole and managing the contracts passed with individual companies.
Ailleret’s declaration to the Conseil Économique et Social (1963). Ailleret’s post was called directeur général adjoint.
Jean Cabanius, quoted in Picard, Beltran, and Bungener (1985, 191). EDF’s Direction de l’Équipement, the division in charge of building the reactors, had already espoused this working method while developing the hydroelectric sites.
Interviews (21). Taranger had in fact pushed very hard for private industry to take the role of industrial architect and was furious when the EDF engineers rejected this course of action. The animosity left over from this meeting did much to increase the tension between the two institutions.
In order to expedite the development of EDF1’s design, Ailleret formed a Comité Nucléaire within EDF. This committee met about once a month to discuss technological problems associated with reactor design as well as EDF’s overall nuclear policy. It was in the course of these meetings that EDF engineers agreed on the basic characteristics for EDF1.
“Étude des réacteurs énergétiques EDF, projet d’organisation dans le cas d’un réacteur du type uranium naturel-graphite-CO2” (1957a).
The minutes of the EDF-CEA meeting of March 16, 1955, read, “Mr. Guillaumat feels that it is essential that an original French technology exist. But Mr. Gaspard [president of EDF] does not want to play the role of patron that much.” Quoted in Lamiral (1988, 29).
They had considered various other possibilities before hitting on this one, including one which combined steel and prestressed concrete. Interviews (fn. 21).
Starting up any gas-graphite reactor for the first time inevitably requires that some slugs be removed before they are fully irradiated, thereby generating what was known as “fatal plutonium.” Thus, the CEA did recover some plutonium from spent EDF1 slugs, but only a minimal amount. The point remains that EDF1 was not designed to produce plutonium. Later EDF reactors, however, did have loading devices that functioned while the reactor was on line. This was not solely a matter of the CEA imposing its will; EDF engineers gradually became convinced that such a system also benefited the economics of running a nuclear power plant. That, however, is a separate story which I treat elsewhere.
Interviews made it quite clear that simplicity of design was a major goal for EDF engineers. It should be noted here that while EDF engineers strove for low cost in designing EDFl, they did not attain that goal, in part because of an unforeseen event: the spherical steel containment vessel cracked, and repairing the crack added tremendous costs and delays to the project. But EDFl represented a first step toward optimizing reactor costs, and the general goal of minimizing costs held throughout EDF’s reactor program.