In late December 1938 Lise Meitner met her nephew Otto Robert Frisch in Kungälv, north of Gøteborg, Sweden, to spend the Christmas holidays with Swedish friends. They had not planned to meet in that unlikely place; both were victims of Nazi persecution: Frisch had been dismissed from his position in Hamburg after the promulgation of the Nazi Civil Service Law on 7 April 1933, and after a year in London he found a haven in the fall of 1934 in Niels Bohr’s Institute for Theoretical Physics in Copenhagen (Frisch 1979a; 1979b, 41–108; Peierls 1981, 285–290). Meitner, protected by her Austrian citizenship, had remained in Berlin until mid-July 1938, four months after the Anschluss of Austria, when she was spirited out of Berlin and into The Netherlands, from whence she flew to Copenhagen and then went on to Stockholm (Sime 1990; 1996, 184–209). Thus, Meitner traveled to Kungälv from Stockholm, and Frisch from Copenhagen.
Both Meitner and Frisch had deep personal worries on their minds: Meitner was fifty-nine years old and had a distinguished scientific reputation, but Manne Siegbahn gave her no financial or personal support in Stockholm to continue her researches. Frisch’s father, a lawyer employed by the publishing firm of Bermann-Fischer in Vienna, had been dismissed from his position in June 1938, and now in December was incarcerated in Dachau, while his mother was threatened and worried sick in Vienna.1
Yet, when aunt and nephew first met at breakfast in their hotel in Kungälv, probably on the morning of 24 December 1938, it was not these deep personal worries that commanded their attention, but a letter that Meitner had just received from Otto Hahn in Berlin in which he reported that he and Fritz Strassmann, in continuing the experiments they had begun with Meitner, had found barium as one of the products of the reaction when uranium was bombarded with neutrons.2 Pondering Hahn’s letter, first in their hotel, then on a hike outdoors in the snow, it suddenly occurred to Meitner and Frisch that the liquid-drop model of the nucleus might provide the key to understanding Hahn and Strassmann’s experiments. Rough calculations substantiated their conjecture, and on 1 January 1939, filled with excitement, Meitner returned to Stockholm and Frisch to Copenhagen.
Two days later, Frisch caught Bohr and told him of his and Meitner’s interpretation. Bohr was greatly surprised. Much later, Frisch said that Bohr burst out with the words, “Oh, what fools we have been! We ought to have seen that before” (Frisch 1967b, 47).
Now, Bohr’s surprise must be taken seriously, because it is precisely such a moment of surprise that reveals that a great transformation in thought has taken place. We recall Arthur Koestler’s analysis of the act of creation in which he argued that the essence of the creative act is the synthesis of two previously unrelated “matrices of thought” (Koestler 1964, 207). Koestler also noted that:
[The] more original a discovery the more obvious it seems afterwards. The creative act is not an act of creation in the sense of the Old Testament. It does not create something out of nothing; it uncovers, selects, re-shuffles, combines, synthesizes already existing facts, ideas, faculties, skills. (Koestler 1964, 120)
Koestler, of course, was mainly concerned with the creative acts of individuals; I think, however, that we shall see that his analysis also seems applicable to the creative act of the two physicists, Meitner and Frisch, at the end of 1938.3
We therefore are presented with several historical questions: Why was Meitner and Frisch’s interpretation so obvious to Bohr as soon as he learned about it? Why was Bohr so astonished that he, or for that matter, other contemporary physicists had failed to see what Meitner and Frisch had seen? Conversely, why were Meitner and Frisch able to achieve their insight, their creative act? To answer these questions, we must examine the history of the liquid-drop model of the nucleus in some detail. We will see that by December 1938 that model was exactly ten years old, and that it had been developed in two distinct phases, from 1928 to 1935 and from 1936 to 1937. Moreover, as we will see immediately, the inventor of the liquid-drop model was not Niels Bohr, as many physicists believe today, but rather George Gamow.
9.1 The Birth of the Liquid-Drop Model
In June 1928 twenty-four-year-old George Gamow left Leningrad to spend the summer in Max Born’s institute in Göttingen where immediately after his arrival he made his first major contribution to nuclear physics, his quantum-mechanical theory of alpha decay (Gamow 1928; Stuewer 1986).4 The following month he wrote to Niels Bohr, making arrangements to visit Copenhagen before returning to Leningrad in the fall.5 He arrived in Copenhagen in September, and when he told Bohr about his theory Bohr was so impressed with it, and with Gamow personally, that he offered Gamow a fellowship to enable him to spend the entire academic year 1928–1929 in his institute. Bohr also arranged for Gamow to visit the Cavendish Laboratory in Cambridge, England, for around five weeks, from early January to mid-February 1929. Gamow thrived in both places. In particular, just before leaving Copenhagen for Cambridge, he invented the liquid-drop model of the nucleus, which he presented for the first time on 7 February 1929, at a meeting of the Royal Society in London to which Ernest Rutherford had invited him.6
Gamow’s basic idea was that the nucleus consists of a collection of
alpha particles with short-range attractive forces between them that balance their Coulomb repulsion. All are in a state of lowest energy since they obey Bose-Einstein statistics. The alpha particles exert an outward pressure owing to their kinetic and potential energy and are held inside the nucleus by its “surface tension,” so one can calculate the total “drop energy”
of the nucleus in terms of the number
of alpha particles inside it, that is, in terms of the atomic weight of the nucleus.
Gamow returned to Russia that summer and in the fall was back in Cambridge on a Rockefeller Foundation fellowship where he pursued his liquid-drop model further during the academic year 1929–1930. The result was a paper, “Mass Defect Curve and Nuclear Constitution” (Gamow 1930), which Rutherford communicated to the Proceedings of the Royal Society on 28 January 1930. In it Gamow developed his liquid-drop model quantitatively.
Gamow’s basic idea was the same as before. He assumed that the nucleus consists of
alpha particles, each of mass
, charge
, kinetic energy
, and potential energy
. He estimated
from Heisenberg’s uncertainty principle and determined
from the virial theorem, finding that
, where
is Planck’s constant and
is the radius of the nucleus. Then, in a separate calculation, he equated the surface tension
to the internal pressure
, both of which are functions of
and
, and found that
, where
cm. The total internal energy
of the alpha particles thus is
. The Coulomb repulsive energy
of the alpha particles at the nuclear surface is
. The total energy
of the nucleus therefore is
, or the sum of an attractive term varying as
and a repulsive term varying as
. Fig. (9.1) is his plot of
versus
, where the shading of the curve indicates the approximate nature of his calculation. Since it was in poor agreement with Francis W. Aston’s mass-defect measurements (dots in the picture), he then took account of the electrons that everyone assumed were present in nuclei and found that the minimum in the mass-defect curve moved out to higher atomic weights as Aston’s measurements required.
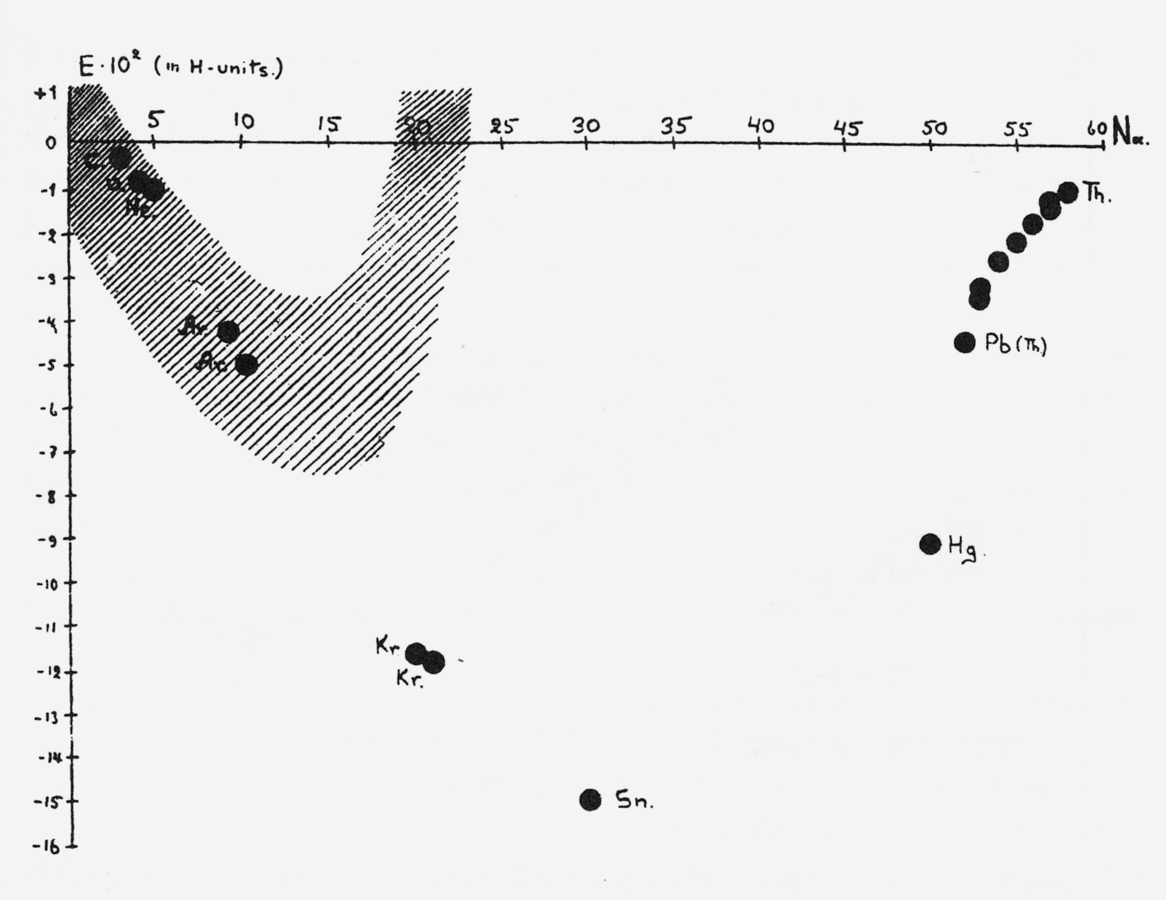




Fig. 9.1: Gamow’s plot of the total nuclear energy
(
in hydrogen-mass units) versus the number of alpha particles
(
in Gamow’s notation) in nuclei. Source: (Gamow 1930, 637).
During the following academic year 1930–1931 Gamow was back in Copenhagen, where he made no further progress on his mass-defect calculations. He again returned to Russia in the summer to renew his passport so that he could attend an international conference on nuclear physics—the first of its kind—that Enrico Fermi was organizing in Rome for October 1931.7 The Soviet authorities, however, refused to renew his passport, so he remained in Leningrad where he taught physics, married, and made plans to escape. He thus was in Leningrad when the field of nuclear physics was fundamentally transformed between the end of 1931 and the end of 1932 by the discoveries of deuterium, the neutron, and the positron, and the construction of the Cockcroft-Walton accelerator and the cyclotron.
9.2 The Extension of the Liquid-Drop Model:
Heisenberg and von Weizsäcker
The most important of these developments for our purpose was James Chadwick’s discovery of the neutron in February 1932 (Chadwick 1932a; 1932b), because a few months later, between June and December 1932, Werner Heisenberg submitted for publication his seminal theory of nuclear structure in which he assumed that a neutron and a proton in the nucleus are bound together by exchanging an electron between them (Heisenberg 1932a; 1932b; 1933a). Then, in early 1933, Ettore Majorana, while visiting Heisenberg’s institute in Leipzig, published, on Heisenberg’s urging, a new neutron-proton force involving the exchange of both charge and spin (Majorana 1933). A compelling argument in its favor was that it saturated at the alpha particle, a highly stable particle, whereas Heisenberg’s force saturated at the deuteron, a much less stable particle. Heisenberg therefore adopted Majorana’s exchange force and recalculated the total energy
of a nucleus in terms of the total number of neutrons
and protons
in it. He presented his calculation and a plot of the resulting mass-defect curve, see fig. (9.2), at the seventh Solvay Congress in Brussels in October 1933 (Heisenberg 1933b). He found, just as Gamow had, that the mass-defect curve has a minimum in it at nuclei of intermediate atomic weights. That must have been particularly gratifying to Gamow, who was present at the Solvay Congress: Quite mysteriously, both he and his wife were given passports and visas to attend it—an opportunity they took to leave the Soviet Union for good (Gamow 1970, 118–133).
Two years later, in 1935, Heisenberg’s student Carl Friedrich von Weizsäcker built on his mentor’s work and extended Gamow’s liquid-drop model still further by introducing his famous semi-empirical mass formula (von Weizsäcker 1935). The result was the same, namely, a minimum in the mass-defect curve at nuclei of intermediate atomic weights,8 see fig. (9.3). Von Weizsäcker’s semi-empirical mass formula thus represented, historically speaking, the culmination of the line of development that Gamow inaugurated when he invented the liquid-drop model in 1928.
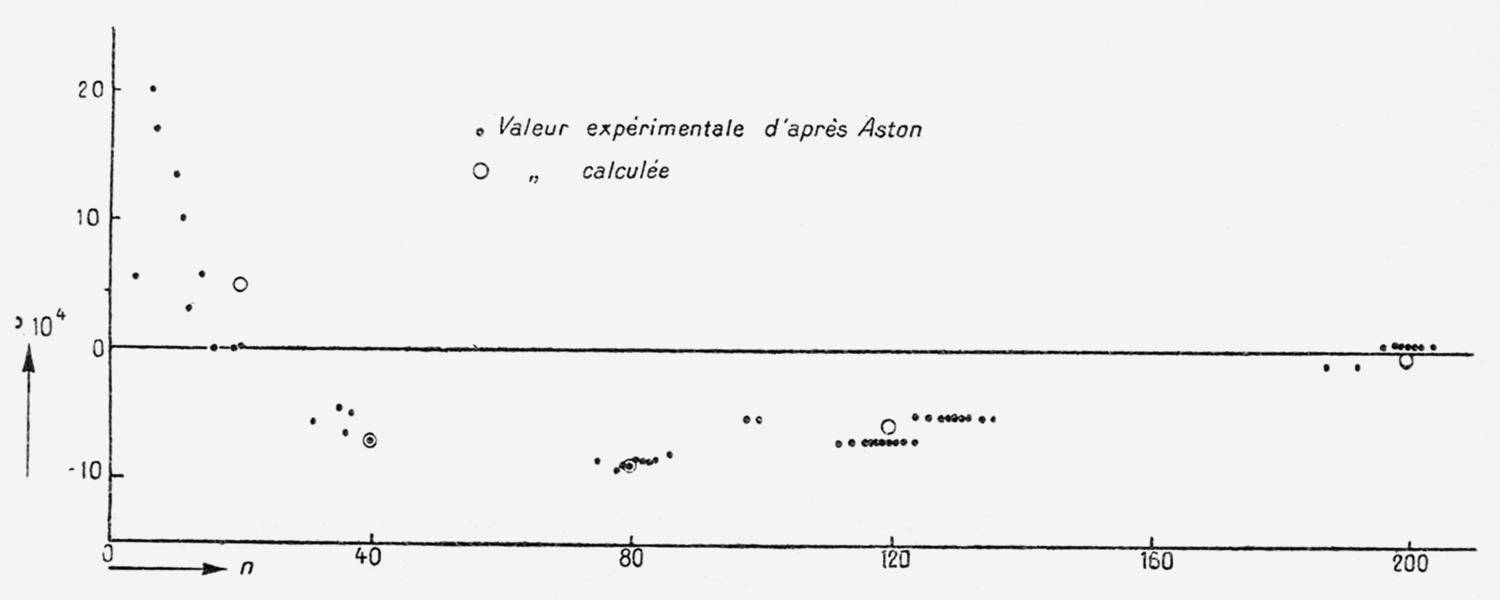




Fig. 9.2: Heisenberg’s plot of the total nuclear energy
(
in units of
) versus the total number of neutrons and protons
in nuclei. The dots represent Aston’s experimental mass-defect data, the circles Heisenberg’s theoretically calculated values. Source: (Heisenberg 1933b, 318).
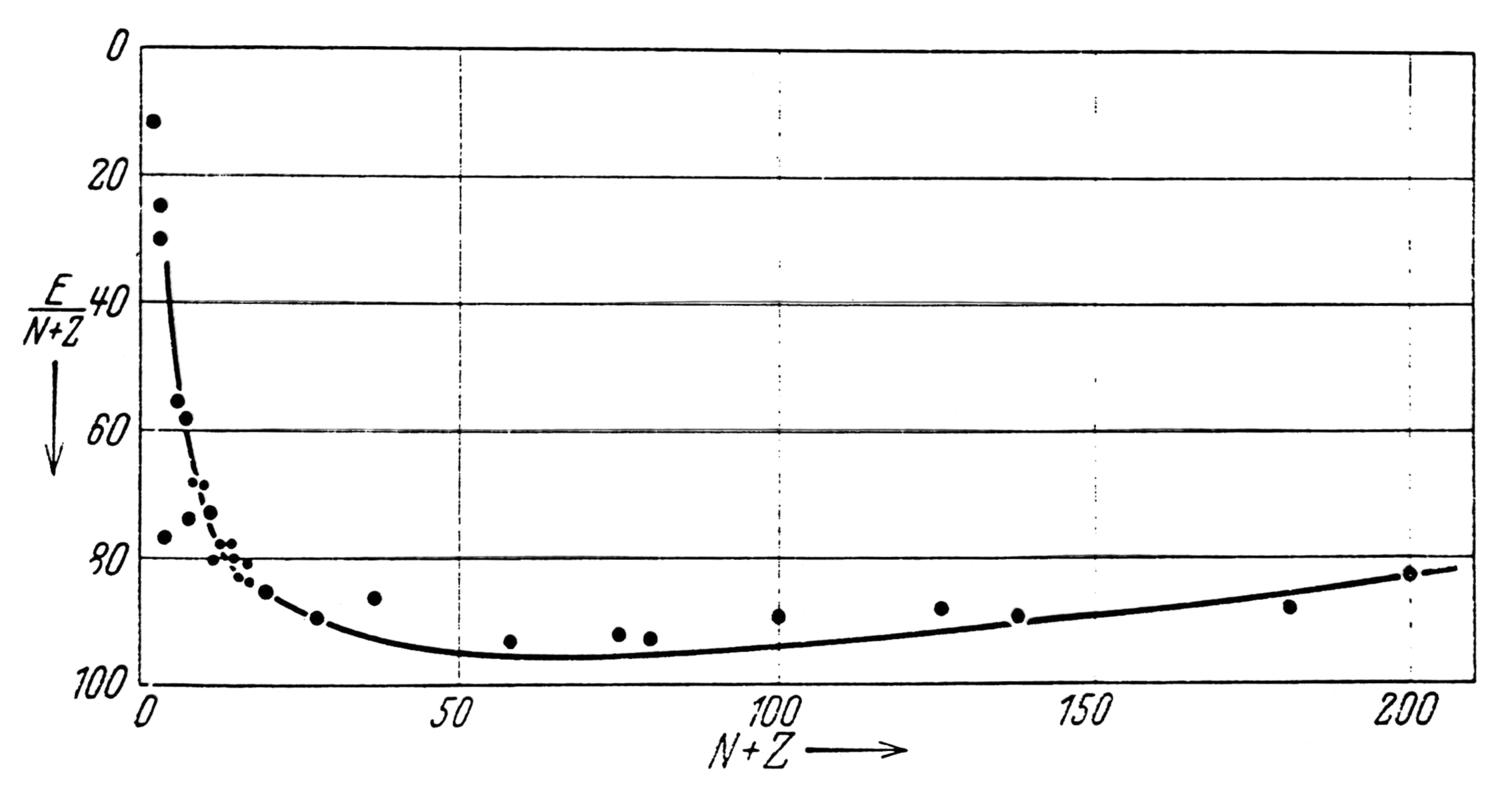



Fig. 9.3: Von Weizsäcker’s plot of the mass defect or energy per nucleon
versus the total number of nucleons (
neutrons +
protons) in nuclei. Source: (von Weizsäcker 1935, 457).
9.3 Bohr’s Theory of the Compound Nucleus
A second phase in the history of liquid-drop model began in February 1936 when Niels Bohr published his theory of the compound nucleus (Bohr 1936). The greatest influence on Bohr’s thought was Enrico Fermi’s discovery in October 1934 that slow neutrons are much more efficacious in producing nuclear reactions than fast ones (Fermi et.al. 1934), contrary to what might be expected on simple energy considerations. The basic idea that Bohr presented was that an incident neutron, for example, interacts with many neutrons and protons in a target nucleus, producing an excited long-lived compound nucleus, which then decays by the emission of a proton, neutron, gamma ray, or by any process that is consistent with conservation of energy.
For our purposes, the principal point of interest is Bohr’s picture of what would happen if the energy of the incident neutron increased more and more:
Even if we could experiment with neutrons or protons of energies of more than a hundred million volts, we should still expect that the excess energy of such particles […] would in the first place be divided among the nuclear particles […]. [We] may, however, in such cases expect that in general not one but several charged or uncharged particles will eventually leave the nucleus as a result of the encounter. For still more violent impacts, with particles of energies of about a thousand million volts, we must even be prepared for the collision to lead to an explosion of the whole nucleus. (Bohr 1936, 348)
Thus, first one, then two, and eventually all of the particles would be exploded out of the target nucleus.
Bohr also concluded that nuclear excitations, in general, were due to oscillations of the surface of a nucleus, not to oscillations of its volume, much like those of an elastic solid. He discussed these ideas widely in lectures on a trip around the world in the first half of 1937 with the help of a picture, see fig. (9.4), drawn for him by Otto Robert Frisch. We see that an incident neutron transfers energy to the target nucleus, causing the surface of the compound nucleus to oscillate and the temperature of the nucleus to rise, after which a single particle is emitted and the nucleus cools down, and finally returns to its initial state and temperature by emitting a gamma ray. He published these ideas in a paper of October 1937 that he wrote with his young assistant, Fritz Kalckar (1937).
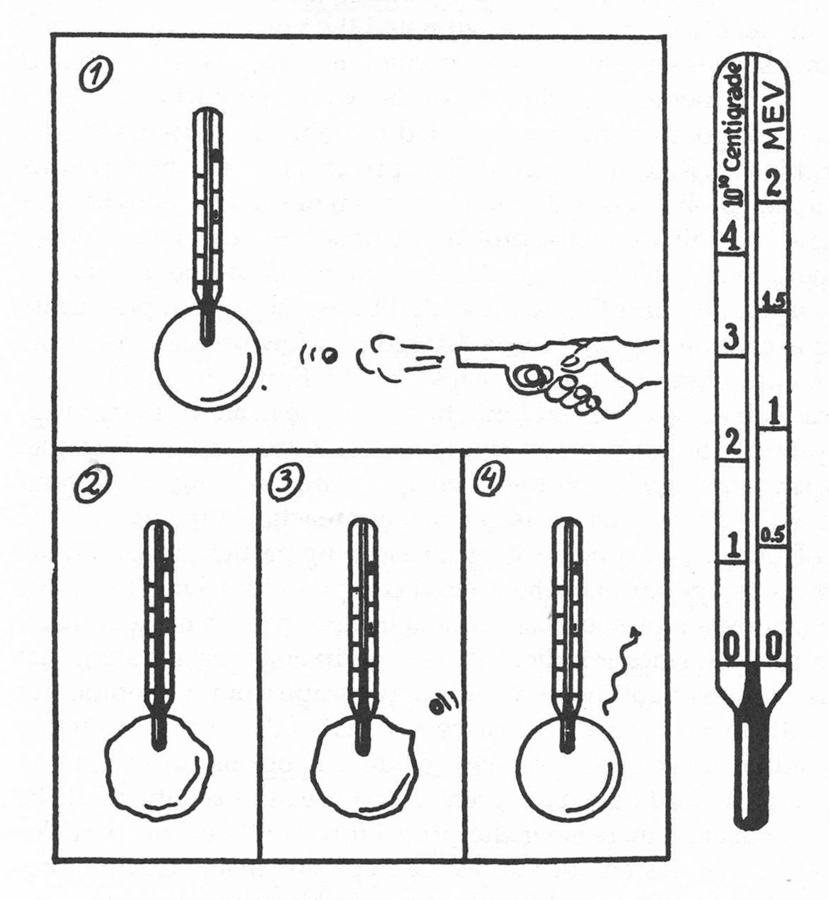
Fig. 9.4: Bohr’s illustration (as drawn by Frisch) of the excitation and deexcitation of a heavy nucleus as absorption and evaporation processes. Source: (Bohr 1937, 163).
9.4 The Interpretation of Fission
We have seen that there were two stages in the development of the liquid-drop model: Stage I, from 1928 to 1935, was delineated by the work of Gamow (1928–1931), Heisenberg (1933), and von Weizsäcker (1935), who applied the liquid-drop model to a calculation of the nuclear mass-defect curve. Their focus, in other words, was on static features of the model. Stage II, from 1936 to 1937, was delineated by the work of Bohr (1936) and Bohr and Kalckar (1937), who applied the liquid-drop model to a calculation of nuclear excitations. Their focus, in other words, was on dynamic features of the model.
These two stages formed what I might call the Berlin and Copenhagen traditions of research on the liquid-drop model, both of which persisted into 1938. Thus, in April 1938 von Weizsäcker published a paper that was largely devoted to a discussion of the liquid-drop model and its application to the calculation of nuclear mass defects (von Weizsäcker 1938). And in August 1938 Bohr presented a report at a meeting of the British Association for the Advancement of Science in Cambridge that concentrated on the problem of nuclear excitations,9 analyzing them as he had in his paper with Kalckar.
Now, just between these two dates, on 13 July 1938, Lise Meitner was spirited out of Berlin and into The Netherlands and then went on to Copenhagen and Stockholm. She was thoroughly embedded in the Berlin tradition, being completely familiar with both Heisenberg’s and von Weizsäcker’s work. Thus, she was present at the seventh Solvay Congress in October 1933 where she heard Heisenberg outline his calculation of the nuclear mass-defect curve and saw its agreement with Aston’s experimental data, and she came into close contact with von Weizsäcker when, after completing his Habilitationsschrift under Heisenberg in Leipzig (von Weizsäcker 1936), he moved to Berlin in July 1936.
Similarly, Otto Robert Frisch was thoroughly embedded in the Copenhagen tradition. He was present in Bohr’s institute when Bohr conceived his theory of the compound nucleus, and he immediately carried out experiments related to Bohr’s theory (Frisch 1937). Moreover, as I noted above, he even drew the pictures that Bohr used to illustrate his theory in his lectures in Europe and on his trip around the world during the first half of 1937.
It was these two research traditions, then, that came together for the first time in the minds of Meitner and Frisch, probably on the morning of 24 December 1938, to produce an entirely new application of the liquid-drop model, their momentous interpretation of nuclear fission. I have reconstructed their conversation based upon Frisch’s several recollections of their memorable walk in the snow in Kungälv, Sweden (Frisch 1967a, 144–145; 1967b, 47; 1973; 1979a, 71; 1979b, 114–116).10
Meitner rejected the idea that Hahn had made a mistake; he was too good a chemist to have done so: When he said that he and Strassmann had found barium when neutrons bombarded uranium, they had found barium.
Both Meitner and Frisch realized that this could not result from a chipping off or cracking up of the uranium nucleus.
Meitner then seems to have thought of the liquid-drop model of the nucleus in this connection, and she made a sketch of a large circle with a smaller circle inside it.
Frisch immediately interpreted Meitner’s sketch as an end-on view of a dumbbell—as an elongated liquid drop with a constriction between its two halves.
Meitner then estimated the amount of energy that would be produced if the uranium nucleus would split into two nuclei at the middle of the periodic table, finding it to be about 200 MeV—an enormous amount. As Frisch remarked, she “had the mass-defect curve pretty well in her head” (Frisch 1967b, 47).
Meanwhile, Frisch had estimated that the electric charge of a nucleus would diminish its surface tension to around zero for a nucleus of nuclear charge
, and he also calculated that two nuclei in the middle of the periodic table, if initially in contact, would fly apart under their mutual Coulomb repulsion with an energy of about 200 MeV—in agreement with Meitner’s figure.
As Frisch said, “We put our different kinds of knowledge together” (Frisch 1973, 833). In other words, as Koestler would have claimed, a synthesis of Meitner’s and Frisch’s different “matrices of thought” occurred.
9.5 Aftermath
We now can understand, I think, Bohr’s great surprise when Frisch told Bohr of Meitner’s and his interpretation of Hahn and Strassmann’s experiments in Copenhagen on 3 January 1939, and why Bohr had not seen what they now saw. Thus, when Bohr presented his theory of the compound nucleus in February 1936 his picture of the disintegration of a heavy nucleus when bombarded with neutrons was a completely different one: With ever increasing neutron energy first one, then two, then an increasing number of neutrons and protons would be expelled from the compound nucleus until eventually, at very high neutron energies, it would explode, sending its neutrons and protons out in all directions. Moreover, in his and Kalckar’s paper of October 1937, Bohr was inclined to view the nucleus as an elastic solid, not as a liquid drop, when considering its surface oscillations and excitations. Perhaps most fundamentally, however, since Bohr was primarily interested in nuclear reactions and excitations, he never seems to have incorporated Gamow’s, Heisenberg’s, and von Weizsäcker’s mass-defect calculations into his thinking. He never seems to have appreciated the compensatory roles played by the repulsive surface charge and attractive surface tension in delicately maintaining the stability of a heavy nucleus like uranium.
Now, however, given Meitner and Frisch’s interpretation, Bohr immediately understood it and discussed it with Frisch over a period of four days before he boarded a ship for the United States on 7 January 1939, where immediately after his arrival in New York on 16 January his traveling companion, Léon Rosenfeld, let the cat out of the bag in Princeton, and where Bohr and Fermi announced the discovery and interpretation of nuclear fission at the fifth Conference on Theoretical Physics at George Washington University in Washington, D.C., on 26 January 1939 (Stuewer 1985), after which the news spread rapidly from coast to coast in the United States. Bohr then went on to Princeton where he became completely absorbed in the fission process, and where he and John Archibald Wheeler produced their classic paper, “The Mechanism of Nuclear Fission” (Bohr and Wheeler 1939).
Meanwhile, in Copenhagen, Frisch began to carry out experiments and around 3:00 A.M. on the morning of Friday, 13 January 1939, he first detected the fission fragments from uranium (Frisch 1939). He recalled that four hours later the postman woke him up and handed him a telegram from his mother saying that his father had been released from Dachau, and that both of his parents now could emigrate to Sweden (Frisch 1973, 833).11
9.6 Conclusion
By early 1939 the history of the liquid-drop model had become obscured, because Bohr, in his and Kalckar’s paper of October 1937, had failed to cite Gamow as its creator, even though Gamow had conceived it in Bohr’s institute in December 1928, perhaps because Bohr saw his application of it as being so different from Gamow’s. And Bohr’s omission was immediately propagated in the literature. Hans A. Bethe, in the second part of his famous three-part article in the Reviews of Modern Physics (the Bethe Bible) of April 1937, based his discussion of the liquid-drop model on Bohr and Kalckar’s paper, which he read in its pre-publication form when he met Bohr in the United States during his trip around the world. Consequently, Bethe unwittingly and ironically stated in his first sentence that: “It was pointed out by Bohr and Kalckar (B33) that a nucleus should be considered as a drop of liquid […]” (Bethe 1937, 86, 170).12 Moreover, Bohr did not correct his omission of Gamow, or Bethe’s mistake, in his and Wheeler’s paper of 1939.
Gamow himself, however, never forgot his contribution. In January 1968 he prepared what no doubt was his last curriculum vitae (he died on 20 August 1968), and under the section he entitled “Theory of Nuclear Fluid” he noted that in Bohr’s institute he had “proposed a hypothesis that atomic nuclei can be treated as little droplets of so-called ‘nuclear fluid’. These views led ultimately to the present theory of nuclear fission and fusion.”13
Abbreviations and Archives
AHQP | Archive for History of Quantum Physics. American Philosophical Society, Philadelphia |
BSC | Bohr Scientific Correspondence in the AHQP |
Niels Bohr Library & Archives | American Institute of Physics, College Park, MD, USA, http://www.aip.org/history/ohilist/4616.html |
Frisch Papers | Trinity College Archive, University of Cambridge |
Gamow Papers | Manuscript Division, Library of Congress, Washington, D.C. |
Meitner Papers | Churchill College Archive, University of Cambridge |
References
Amaldi, Edoardo, Enrico Persico, E. P., Rasetti Enrico (1962). Enrico Fermi. Collected Papers (Note e Memorie). Vol. 1. . Chicago: The University of Chicago Press.
Bethe, Hans A. (1937). Nuclear Physics. B. Nuclear Dynamics, Theoretical. Reviews of Modern Physics 9: 69-244
Bethe, Hans A., Robert F. Bacher, R.F. B. (1986). Basic Bethe. Seminal Articles on Nuclear Physics, 1936–1937. New York: Tomash PublishersAmerican Institute of Physics.
Beyer, Robert T. (1949). Foundations of Nuclear Physics. Facsimiles of Thirteen Fundamental Studies as They Were Originally Reported in the Scientific Journals. New York: Dover.
Bohr, Niels (1936). Neutron Capture and Nuclear Constitution. Nature 137: 344-388
- (1937). Transmutation of Atomic Nuclei. Science 86: 161-165
Bohr, Niels, Fritz Kalckar (1937). On the Transmutation of Atomic Nuclei by Impact of Material Particles. I. General Theoretical Remarks. Det Kongelige Danske Videnskabernes Selskab. Mathematisk-fysiske Meddelelser 14(10): 1-40
Bohr, Niels, John A. Wheeler (1939). The Mechanism of Nuclear Fission. Physical Review 56: 426-450
Chadwick, James (1932a). Possible Existence of a Neutron. Nature 129: 312
- (1932b). The Existence of a Neutron. Proceedings of the Royal Society A 136: 692-708
Fermi, Enrico, Edoardo Amaldi, E. A., Pontecorvo Edoardo, P. E. (1934). Azione di sostanze idrogenate sulla radioattività provocata da neutroni. I.. La Ricerca Scientifica 5: 282-283
Frisch, Otto R. (1937). On the Selective Capture of Slow Neutrons. Det Kongelige Danske Videnskabernes Selskab. Mathematisk-fysiske Meddelelser 14(12): 1-31
- (1939). Physical Evidence for the Division of Heavy Nuclei under Neutron Bombardment. Nature 143: 276
- (1967a). The Interest is Focussing on the Atomic Nucleus. In: Niels Bohr: His Life and Work as Seen by His Friends and Colleagues Ed. by Stefan Rozental. Amsterdam: Wiley 137-148
- (1967b). How It All Began. Physics Today 20(11): 43-48
- (1973). Walk in the Snow. New Scientist 60: 833
- (1979a). Experimental Work with Nuclei: Hamburg, London, Copenhagen. In: Nuclear Physics in Retrospect: Proceedings of a Symposium on the 1930s Ed. by Roger H. Stuewer. Minneapolis: University of Minnesota Press 65-75
- (1979b). What Little I Remember. Cambridge: Cambridge University Press.
Gamow, George (1928). Zur Quantentheorie des Atomkernes. Zeitschrift für Physik 51: 204-212
- (1930). Mass Defect Curve and Nuclear Constitution. Proceedings of the Royal Society A 126: 632-644
- (1970). My World Line: An Informal Autobiography. New York: Viking.
Gurney, Ronald W., Edward U. Condon (1928). Wave Mechanics and Radioactive Disintegration. Nature 122: 439
- (1929). Quantum Mechanics and Radioactive Disintegration. Physical Review 33: 127-140
Heisenberg, Werner (1932a). Über den Bau der Atomkerne I. Zeitschrift für Physik 77: 1-11
- (1932b). Über den Bau der Atomkerne II. Zeitschrift für Physik 78: 156-164
- (1933a). Über den Bau der Atomkerne III. Zeitschrift für Physik 80: 587-596
- (1933b). Considérations théoriques générales sur la structure du noyau. In: Structure et propriétés des noyaux atomiques. Rapports et discussions du septième conseil de physique tenu à Bruxelles du 22 au 29 octobre 1933 Ed. by Institut International Physique Solvay. Paris: Gauthier-Villars 289-323
Institut International de Physique Solvay (1934). Structure et propriétés des noyaux atomiques. Rapports et discussions du septième conseil de physique tenu à Bruxelles du 22 au 29 octobre 1933. Paris: Gauthier-Villars.
Koestler, Arthur (1964). The Act of Creation. New York: Macmillan.
Majorana, Ettore (1933). Über die Kerntheorie. Zeitschrift für Physik 82: 137-145
Meitner, Lise, Otto R. Frisch (1939). Disintegration of Uranium by Neutrons: A New Type of Nuclear Reaction. Nature 143: 239-240
Nuclear Physics (1938). Nature Supplement..
Peierls, Rudolf (1981). Otto Robert Frisch. 1 October 1904–22 September 1979. Biographical Memoirs of Fellows of the Royal Society of London 27: 283-306
- (1986). Niels Bohr Collected Works. Amsterdam: Elsevier.
Reale Accademia d ' Italia (1932). Convegno di Fisica Nucleare, Ottobre 1931–XI. Roma: Reale Accademia d'Italia.
Rozental, Stefan (1967). Niels Bohr: His Life and Work as Seen by His Friends and Colleagues. Amsterdam: Wiley.
Rutherford, Ernest F., Francis W. Aston, F.W. A., Chadwick Francis W., C. F.W., James Chadwick, J. C., Ellis James (1929). Discussion on the Structure of Atomic Nuclei. Proceedings of the Royal Society A 123: 262-267
Sime, Ruth Lewin (1990). Lise Meitner's Escape from Germany. American Journal of Physics 58: 262-267
- (1996). Lise Meitner: A Life in Physics. Berkeley: University of California Press.
Stuewer, Roger H. (1979). Nuclear Physics in Retrospect: Proceedings of a Symposium on the 1930s. Minneapolis: University of Minnesota Press.
- (1985). Bringing the News of Fission to America. Physics Today 38(10): 2-10
- (1986). Gamow's Theory of Alpha-Decay. In: The Kaleidoscope of Science Ed. by Edna Ullmann-Margalit. Dordrecht: D. Reidel 147-186
- (1994). The Origin of the Liquid-Drop Model and the Interpretation of Nuclear Fission. Perspectives on Science 2: 39-92
Ullmann-Margalit, Edna (1986). The Kaleidoscope of Science. Dordrecht: D. Reidel.
von Weizsäcker, Carl Friedrich (1935). Zur Theorie der Kernmassen. Zeitschrift für Physik 96: 431-458
- (1936). Über die Spinabhängigkeit der Kernkräfte. Zeitschrift für Physik 102: 572-602
- (1938). Neuere Modellvorstellungen über den Bau der Atomkerne. Die Naturwissenschaften 26: 209-217
Footnotes
Frisch to Meitner, 1 October 1938, Frisch Papers. See also (Frisch 1973).
Hahn to Meitner, 19 December 1938, Meitner Papers.
For a full account, see (Stuewer 1994).
The same theory was published independently and virtually simultaneously by Ronald W. Gurney and Edward U. Condon; see (Gurney and Condon 1928; 1929).
Gamow to Bohr, 21 July 1928, BSC.
“Discussion on the Structure of Atomic Nuclei” (Rutherford et.al. 1929).
To anticipate: If a heavy nucleus like uranium
were to split up into two nuclei in the middle of the periodic table
, then the difference in their energies per nucleon
, as represented by the difference in their ordinates on the mass-defect curve, would be released in the process.
See also: Interview of Otto Robert Frisch by Charles Weiner on 3 May 1967, Niels Bohr Library & Archives; and (Frisch 1973). Nuclear Pioneer Lecture Honouring Lise Meitner. Presented to the Society of Nuclear Medicine, Miami Beach, Florida, June 1973. File C74, p. 9–10, Frisch Papers; and Frisch, Otto R. (no date). The Origin of Nuclear Fission. File C74, Frisch Papers.
See also: Interview of Otto Robert Frisch by Charles Weiner on 3 May 1967, Niels Bohr Library & Archives; and (Frisch 1973). Nuclear Pioneer Lecture Honouring Lise Meitner. Presented to the Society of Nuclear Medicine, Miami Beach, Florida, June 1973. File C74, p. 13, Frisch Papers.
Bethe revealed his subtle sense of humor in his reference B33, because, knowing Bohr’s propensity for writing many drafts of his papers before releasing them for publication, Bethe gave 1939 as the year of publication of Bohr and Kalckar’s paper.
Curriculum Vitae dated January 1968 and entitled “GAMOW, GEORGE, American Physicist Born March 4, 1904, Odessa, Russia.”, Gamow Papers.